42 Lewis Dot Symbols and Lewis Structures (Writing Lewis Symbols for Atoms)
LumenLearning
Representing Valence Electrons in Lewis Symbols
Lewis symbols use dots to visually represent the valence electrons of an atom.
LEARNING OBJECTIVES
Recall the Lewis structure formalism for representing valance electrons
KEY TAKEAWAYS
Key Points
- Electrons exist outside of an atom ‘s nucleus and are found in principal energy levels that contain only up to a specific number of electrons.
- The outermost principal energy level that contains electrons is called the valence level and contains valence electrons.
- Lewis symbols are diagrams that show the number of valence electrons of a particular element with dots that represent lone pairs.
- Lewis symbols do not visualize the electrons in the inner principal energy levels.
Key Terms
- principal energy levels: The different levels where electrons can be found and that occur at specific distances from the atom’s nucleus. Each level is associated with a particular energy value that electrons within it have.
- valence level: The outermost principal energy level, which is the level furthest away from the nucleus that still contains electrons.
- valence electrons: The electrons of atoms that participate in the formation of chemical bonds.
- Lewis symbols: Symbols of the elements with their number of valence electrons represented as dots
Lewis symbols (also known as Lewis dot diagrams or electron dot diagrams) are diagrams that represent the valence electrons of an atom. Lewis structures (also known as Lewis dot structures or electron dot structures) are diagrams that represent the valence electrons of atoms within a molecule. These Lewis symbols and Lewis structures help visualize the valence electrons of atoms and molecules, whether they exist as lone pairs or within bonds.
Principal Energy Levels
An atom consists of a positively charged nucleus and negatively charged electrons. The electrostatic attraction between them keeps electrons ‘bound’ to the nucleus so they stay within a certain distance of it. Careful investigations have shown that not all electrons within an atom have the same average position or energy. We say the electrons ‘reside’ in different principal energy levels, and these levels exist at different radii from the nucleus and have rules regarding how many electrons they can accommodate.
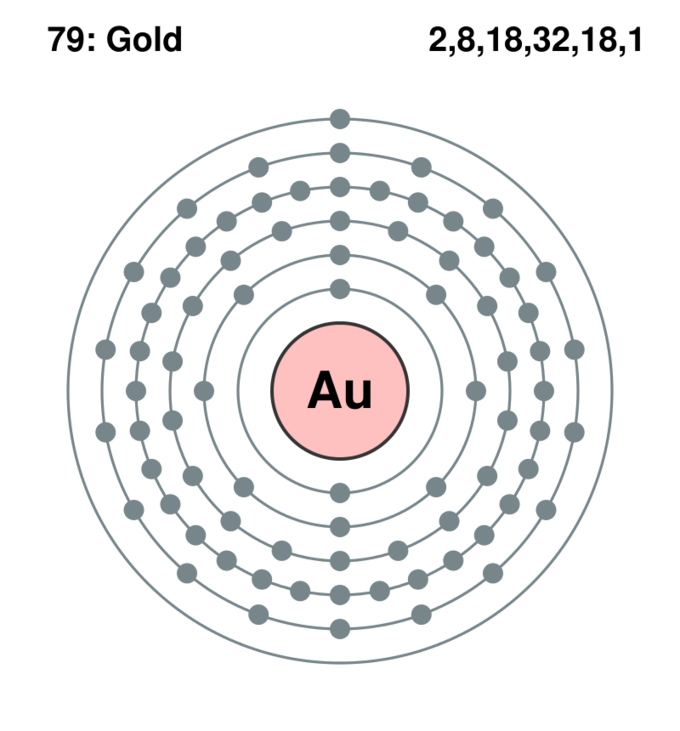
As an example, a neutral atom of gold (Au) contains 79 protons in its nucleus and 79 electrons. The first principal energy level, which is the one closest to the nucleus, can hold a maximum of two electrons. The second principal energy level can have 8, the third can have 18, and so on, until all 79 electrons have been distributed.
The outermost principal energy level is of great interest in chemistry because the electrons it holds are the furthest away from the nucleus, and therefore are the ones most loosely held by its attractive force; the larger the distance between two charged objects, the smaller the force they exert on each other. Chemical reactivity of all of the different elements in the periodic table depends on the number of electrons in that last, outermost level, called the valence level or valence shell. In the case of gold, there is only one valence electron in its valence level.
Octet of Valence Electrons
Atoms gain, lose, or share electrons in their valence level in order to achieve greater stability, or a lower energy state. From this perspective, bonds between atoms form so that the bonded atoms are in a lower energy state compared to when they were by themselves. Atoms can achieve this more stable state by having a valence level which contains as many electrons as it can hold. For the first principal energy level, having two electrons in it is the most stable arrangement, while for all other levels outside of the first, eight electrons are necessary to achieve the most stable state.
Lewis Symbols
In the Lewis symbol for an atom, the chemical symbol of the element (as found on the periodic table) is written, and the valence electrons are represented as dots surrounding it. Only the electrons in the valence level are shown using this notation. For example, the Lewis symbol of carbon depicts a “C’ surrounded by 4 valence electrons because carbon has an electron configuration of 1s22s22p2.

Electrons that are not in the valence level are not shown in the Lewis symbol. The reason for this is that the chemical reactivity of an atom of the element is solely determined by the number of its valence electrons, and not its inner electrons. Lewis symbols for atoms are combined to write Lewis structures for compounds or molecules with bonds between atoms.
Writing Lewis Symbols for Atoms
The Lewis symbol for an atom depicts its valence electrons as dots around the symbol for the element.
LEARNING OBJECTIVES
Write Lewis symbols for atoms
KEY TAKEAWAYS
Key Points
- The columns, or groups, in the periodic table are used to determine the number of valence electrons for each element.
- The noble/ inert gases are chemically stable and have a full valence level of electrons.
- Other elements react in order to achieve the same stability as the noble gases.
- Lewis symbols represent the valence electrons as dots surrounding the elemental symbol for the atom.
Key Terms
- group: A column in the periodic table that consists of elements with similar chemical reactivity, because they have the same number of valence electrons.
- Noble Gases: Inert, or unreactive, elements in the last group in the periodic table which are typically found in the gaseous form.
- Lewis symbol: Formalism in which the valence electrons of an atom are represented as dots.
Determining the Number of Valence Electrons
In order to write the Lewis symbol for an atom, you must first determine the number of valence electrons for that element. The arrangement of the periodic table can help you figure out this information. Since we have established that the number of valence electrons determines the chemical reactivity of an element, the table orders the elements by number of valence electrons.
Each column (or group) of the periodic table contains elements that have the same number of valence electrons. Furthermore, the number of columns (or groups) from the left edge of the table tells us the exact number of valence electrons for that element. Recall that any valence level can have up to eight electrons, except for the first principal energy level, which can only have two.
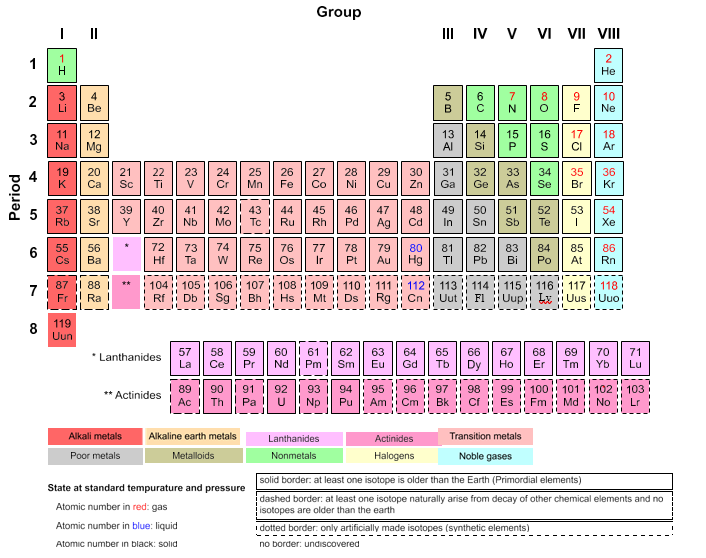
Some periodic tables list the group numbers in Arabic numbers instead of Roman numerals. In that case, the transition metal groups are included in the counting and the groups indicated at the top of the periodic table have numbers 1, 2, 13, 14, 15, 16, 17, 18. The corresponding roman numerals used are I, II, III, IV, V, VI, VII, VIII.
Survey of the Groups in the Periodic Table
Take the first column or group of the periodic table (labeled ‘I’): hydrogen (H), lithium (Li), sodium (Na), potassium (K), etc. Each of these elements has one valence electron. The second column or group (labeled ‘II’) means that beryllium (Be), magnesium (Mg), calcium (Ca), etc., all have two valence electrons.
The middle part of the periodic table that contains the transition metals is skipped in this process for reasons having to do with the electronic configuration of these elements.
Proceeding to the column labeled ‘III’, we find that those elements (B, Al, Ga, In,…) have three valence electrons in their outermost or valence level.
We can continue this inspection of the groups until we reach the eighth and final column, in which the most stable elements are listed. These are all gaseous under normal conditions of temperature and pressure, and are called ‘noble gases.’ Neon (Ne), argon (Ar), krypton (Kr), etc., each contain eight electrons in their valence level. Therefore, these elements have a full valence level that has the maximum number of electrons possible. Helium (He), at the very top of this column is an exception because it has two valence electrons; its valence level is the first principal energy level which can only have two electrons, so it has the maximum number of electrons in its valence level as well.
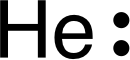
The noble gases represent elements of such stability that they are not chemically reactive, so they can be called inert. In other words, they don’t need to bond with any other elements in order to attain a lower energy configuration. We explain this phenomenon by attributing their stability to having a ‘full’ valence level.
The significance in understanding the nature of the stability of noble gases is that it guides us in predicting how other elements will react in order to achieve the same electronic configuration as the noble gases by having a full valence level.
Writing Lewis Symbols for Atoms
Lewis symbols for the elements depict the number of valence electrons as dots. In accordance with what we discussed above, here are the Lewis symbols for the first twenty elements in the periodic table. The heavier elements will follow the same trends depending on their group.
Once you can draw a Lewis symbol for an atom, you can use the knowledge of Lewis symbols to create Lewis structures for molecules.
Valence Electrons and the Periodic Table: Electrons can inhabit a number of energy shells. Different shells are different distances from the nucleus. The electrons in the outermost electron shell are called valence electrons, and are responsible for many of the chemical properties of an atom. This video will look at how to find the number of valence electrons in an atom depending on its column in the periodic table.
Introduction to Lewis Structures for Covalent Molecules
In covalent molecules, atoms share pairs of electrons in order to achieve a full valence level.
LEARNING OBJECTIVES
Predict and draw the Lewis structure of simple covalent molecules and compounds
KEY TAKEAWAYS
Key Points
- The octet rule says that the noble gas electronic configuration is a particularly favorable one that can be achieved through formation of electron pair bonds between atoms.
- In many atoms, not all of the electron pairs comprising the octet are shared between atoms. These unshared, non-bonding electrons are called ‘ lone pairs ‘ of electrons.
- Although lone pairs are not directly involved in bond formation, they should always be shown in Lewis structures.
- There is a logical procedure that can be followed to draw the Lewis structure of a molecule or compound.
Key Terms
- octet rule: Atoms try to achieve the electronic configuration of the noble gas nearest to them in the periodic table by achieving a full valence level with eight electrons.
- exceptions to the octet rule: Hydrogen (H) and helium (He) only need two electrons to have a full valence level.
- covalent bond: Two atoms share valence electrons in order to achieve a noble gas electronic configuration.
- Lewis structure: Formalism used to show the structure of a molecule or compound, in which shared electrons pairs between atoms are indicated by dashes. Non-bonding, lone pairs of electrons must also be shown.
The Octet Rule
Noble gases like He, Ne, Ar, Kr, etc., are stable because their valence level is filled with as many electrons as possible. Eight electrons fill the valence level for all noble gases, except helium, which has two electrons in its full valence level. Other elements in the periodic table react to form bonds in which valence electrons are exchanged or shared in order to achieve a valence level which is filled, just like in the noble gases. We refer to this chemical tendency of atoms as ‘the octet rule,’ and it guides us in predicting how atoms combine to form molecules and compounds.
Covalent Bonds and Lewis Diagrams of Simple Molecules
The simplest example to consider is hydrogen (H), which is the smallest element in the periodic table with one proton and one electron. Hydrogen can become stable if it achieves a full valence level like the noble gas that is closest to it in the periodic table, helium (He). These are exceptions to the octet rule because they only require 2 electrons to have a full valence level.
Two H atoms can come together and share each of their electrons to create a ‘ covalent bond.’ The shared pair of electrons can be thought of as belonging to either atom, and thus each atom now has two electrons in its valence level, like He. The molecule that results is H2, and it is the most abundant molecule in the universe.
The Lewis formalism used for the H2 molecule is H:H or H—H. The former, known as a ‘Lewis dot diagram,’ indicates a pair of shared electrons between the atomic symbols, while the latter, known as a ‘Lewis structure,’ uses a dash to indicate the pair of shared electrons that form a covalent bond. More complicated molecules are depicted this way as well.
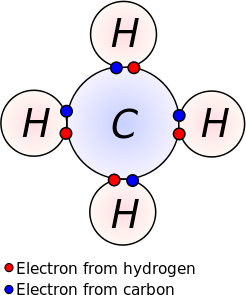
Now consider the case of fluorine (F), which is found in group VII (or 17) of the periodic table. It therefore has 7 valence electrons and only needs 1 more in order to have an octet. One way that this can happen is if two F atoms make a bond, in which each atom provides one electron that can be shared between the two atoms. The resulting molecule that is formed is F2, and its Lewis structure is F—F.
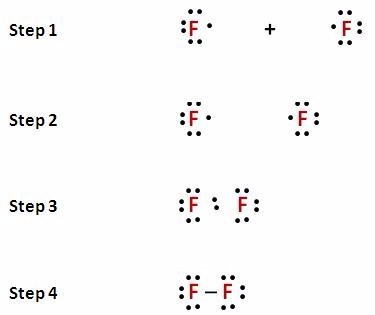
After a bond has formed, each F atom has 6 electrons in its valence level which are not used to form a bond. These non-bonding valence electrons are called ‘lone pairs’ of electrons and should always be indicated in Lewis diagrams.
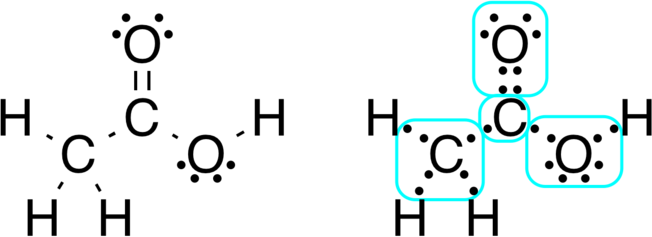
Procedure for Drawing Simple Lewis Structures
We have looked at how to determine Lewis structures for simple molecules. The procedure is as follows:
- Write a structural diagram of the molecule to clearly show which atom is connected to which (although many possibilities exist, we usually pick the element with the most number of possible bonds to be the central atom).
- Draw Lewis symbols of the individual atoms in the molecule.
- Bring the atoms together in a way that places eight electrons around each atom (or two electrons for H, hydrogen) wherever possible.
- Each pair of shared electrons is a covalent bond which can be represented by a dash.
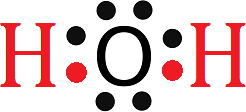
Multiple bonds can also form between elements when two or three pairs of electrons are shared to produce double or triple bonds, respectively. The Lewis structure for carbon dioxide, CO2, is a good example of this.
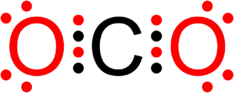
In order to achieve an octet for all three atoms in CO2, two pairs of electrons must be shared between the carbon and each oxygen. Since four electrons are involved in each bond, a double covalent bond is formed. You can see that this is how the octet rule is satisfied for all atoms in this case. When a double bond is formed, you still need to show all electrons, so double dashes between the atoms show that four electrons are shared.

Lewis Structures for Polyatomic Ions
The Lewis structure of an ion is placed in brackets and its charge is written as a superscript outside of the brackets, on the upper right.
LEARNING OBJECTIVES
Apply the rules for drawing Lewis structures to polyatomic ions
KEY TAKEAWAYS
Key Points
- Ions are treated almost the same way as a molecule with no charge. However, the number of electrons must be adjusted to account for the net electric charge of the ion.
- When counting electrons, negative ions should have extra electrons placed in their Lewis structures, while positive ions should have fewer electrons than an uncharged molecule.
Key Terms
- polyatomic ion: A charged species composed of two or more atoms covalently bonded, or of a metal complex that acts as a single unit in acid-base chemistry or in the formation of salts. Also known as a molecular ion.
The total number of electrons represented in a Lewis structure is equal to the sum of the numbers of valence electrons in each individual atom. Non-valence electrons are not represented in Lewis structures. After the total number of available electrons has been determined, electrons must be placed into the structure.
Lewis structures for polyatomic ions are drawn by the same methods that we have already learned. When counting electrons, negative ions should have extra electrons placed in their Lewis structures; positive ions should have fewer electrons than an uncharged molecule. When the Lewis structure of an ion is written, the entire structure is placed in brackets, and the charge is written as a superscript on the upper right, outside of the brackets. For example, consider the ammonium ion, NH4+, which contains 9 (5 from N and 1 from each of the four H atoms) –1 = 8 electrons. One electron is subtracted because the entire molecule has a +1 charge.
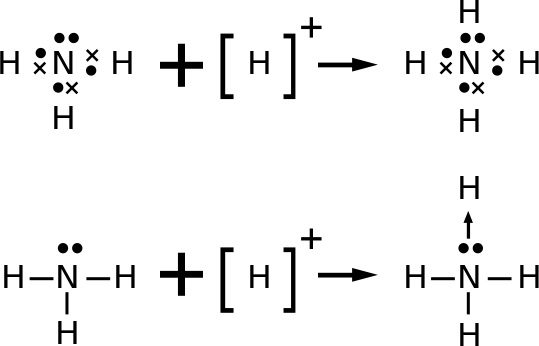
Negative ions follow the same procedure. The chlorite ion, ClO2–, contains 19 (7 from the Cl and 6 from each of the two O atoms) +1 = 20 electrons. One electron is added because the entire molecule has a -1 charge.
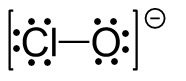
LICENSES AND ATTRIBUTIONS
CC LICENSED CONTENT, SHARED PREVIOUSLY
- Curation and Revision. Provided by: Boundless.com. License: CC BY-SA: Attribution-ShareAlike
CC LICENSED CONTENT, SPECIFIC ATTRIBUTION
- Provided by: African Virtual University. Located at: http://oer.avu.org/bitstream/handle/123456789/42/Introductory%20Chemistry%201.pdf?sequence=4. License: CC BY: Attribution
- Covalent bonding. Provided by: Steve Lower’s Website. Located at: http://www.chem1.com/acad/webtext/chembond/cb03.html#SEC2. License: CC BY-SA: Attribution-ShareAlike
- Valence electron. Provided by: Wikipedia. Located at: http://en.wikipedia.org/wiki/Valence_electron. License: CC BY-SA: Attribution-ShareAlike
- Lewis structure. Provided by: Wikipedia. Located at: http://en.wikipedia.org/wiki/Lewis_structure. License: CC BY-SA: Attribution-ShareAlike
- Lewis structure. Provided by: Wikipedia. Located at: http://en.wikipedia.org/wiki/Lewis_structure. License: CC BY-SA: Attribution-ShareAlike
- valence electrons. Provided by: Wikipedia. Located at: http://en.wikipedia.org/wiki/valence%20electrons. License: CC BY-SA: Attribution-ShareAlike
- Lewis structure. Provided by: Wiktionary. Located at: http://en.wiktionary.org/wiki/Lewis_structure. License: CC BY-SA: Attribution-ShareAlike
- Carbone lewis. Provided by: Wikimedia. Located at: http://en.wikipedia.org/wiki/Lewis_structure%23mediaviewer/File:Carbone_lewis.svg. License:
- Electron shell diagram for Gold. Provided by: Wikimedia. Located at: http://commons.wikimedia.org/wiki/File:Electron_shell_079_Gold.svg. License:
- Provided by: African Virtual University. Located at: http://oer.avu.org/bitstream/handle/123456789/42/Introductory%20Chemistry%201.pdf?sequence=4. License: CC BY: Attribution
- Covalent bonding. Provided by: Steve Lower’s Website. Located at: http://www.chem1.com/acad/webtext/chembond/cb03.html#SEC2. License: CC BY-SA: Attribution-ShareAlike
- Provided by: AskApache. Located at: http://nongnu.askapache.com/fhsst/Chemistry_Grade_10-12.pdf. License:
- Lewis structure. Provided by: Wikipedia. Located at: http://en.wikipedia.org/wiki/Lewis_structure. License: CC BY-SA: Attribution-ShareAlike
- Lewis structure. Provided by: Wiktionary. Located at: http://en.wiktionary.org/wiki/Lewis_structure. License: CC BY-SA: Attribution-ShareAlike
- Carbone lewis. Provided by: Wikimedia. Located at: http://en.wikipedia.org/wiki/Lewis_structure%23mediaviewer/File:Carbone_lewis.svg. License:
- Electron shell diagram for Gold. Provided by: Wikimedia. Located at: http://commons.wikimedia.org/wiki/File:Electron_shell_079_Gold.svg. License:
- Valence Electrons and the Periodic Table. License: . License Terms: Standard YouTube license
- Periodic%20Table%20of%20Elements. Provided by: Wikimedia. Located at: http://commons.wikimedia.org/wiki/Periodic_Table_of_Elements. License: CC BY-SA: Attribution-ShareAlike
- Covalent bonding. Provided by: Steve Lower’s Website. Located at: http://www.chem1.com/acad/webtext/chembond/cb03.html#SEC2. License: CC BY-SA: Attribution-ShareAlike
- octet rule. Provided by: Wiktionary. Located at: http://en.wiktionary.org/wiki/octet_rule. License: CC BY-SA: Attribution-ShareAlike
- Provided by: African Virtual University. Located at: http://oer.avu.org/bitstream/handle/123456789/42/Introductory%20Chemistry%201.pdf?sequence=4. License:
- Carbone lewis. Provided by: Wikimedia. Located at: http://en.wikipedia.org/wiki/Lewis_structure%23mediaviewer/File:Carbone_lewis.svg. License:
- Electron shell diagram for Gold. Provided by: Wikimedia. Located at: http://commons.wikimedia.org/wiki/File:Electron_shell_079_Gold.svg. License:
- Valence Electrons and the Periodic Table. License: . License Terms: Standard YouTube license
- Periodic%20Table%20of%20Elements. Provided by: Wikimedia. Located at: http://commons.wikimedia.org/wiki/Periodic_Table_of_Elements. License: CC BY-SA: Attribution-ShareAlike
- Acqua_Lewis. Provided by: Wikimedia Commons. Located at: http://commons.wikimedia.org/wiki/File:Acqua_Lewis.png. License: CC BY-SA: Attribution-ShareAlike
- Ikatan_kovalen_pada_molekul_F2.jpg. Provided by: Wikimedia. Located at: https://commons.wikimedia.org/wiki/File:Ikatan_kovalen_pada_molekul_F2.jpg. License: CC BY-SA: Attribution-ShareAlike
- Covalent.svg. Provided by: Mikimedia Commons. Located at: http://commons.wikimedia.org/wiki/File:Covalent.svg. License: CC BY-SA: Attribution-ShareAlike
- Carbon-dioxide-octet-Lewis-2D. Provided by: Wikimedia Commons. Located at: http://commons.wikimedia.org/wiki/File:Carbon-dioxide-octet-Lewis-2D.png. License:
- Lewis carbon dioxide. Provided by: Wikimedia Commons. Located at: http://commons.wikimedia.org/wiki/File:Lewis_carbon_dioxide.gif. License:
- Acetic_acid_oxidation_state_analysis.png. Provided by: Wikimedia. Located at: https://commons.wikimedia.org/wiki/File:Acetic_acid_oxidation_state_analysis.png. License:
- polyatomic ion. Provided by: Wikipedia. Located at: http://en.wikipedia.org/wiki/polyatomic%20ion. License: CC BY-SA: Attribution-ShareAlike
- Covalent bonding. Provided by: Steve Lower’s Website. Located at: http://www.chem1.com/acad/webtext/chembond/cb03.html#SEC2. License: CC BY-SA: Attribution-ShareAlike
- Lewis structure. Provided by: Wikipedia. Located at: http://en.wikipedia.org/wiki/Lewis_structure. License: CC BY-SA: Attribution-ShareAlike
- Carbone lewis. Provided by: Wikimedia. Located at: http://en.wikipedia.org/wiki/Lewis_structure%23mediaviewer/File:Carbone_lewis.svg. License:
- Electron shell diagram for Gold. Provided by: Wikimedia. Located at: http://commons.wikimedia.org/wiki/File:Electron_shell_079_Gold.svg. License:
- Valence Electrons and the Periodic Table. License: . License Terms: Standard YouTube license
- Periodic%20Table%20of%20Elements. Provided by: Wikimedia. Located at: http://commons.wikimedia.org/wiki/Periodic_Table_of_Elements. License: CC BY-SA: Attribution-ShareAlike
- Acqua_Lewis. Provided by: Wikimedia Commons. Located at: http://commons.wikimedia.org/wiki/File:Acqua_Lewis.png. License: CC BY-SA: Attribution-ShareAlike
- Ikatan_kovalen_pada_molekul_F2.jpg. Provided by: Wikimedia. Located at: https://commons.wikimedia.org/wiki/File:Ikatan_kovalen_pada_molekul_F2.jpg. License: CC BY-SA: Attribution-ShareAlike
- Covalent.svg. Provided by: Mikimedia Commons. Located at: http://commons.wikimedia.org/wiki/File:Covalent.svg. License: CC BY-SA: Attribution-ShareAlike
- Carbon-dioxide-octet-Lewis-2D. Provided by: Wikimedia Commons. Located at: http://commons.wikimedia.org/wiki/File:Carbon-dioxide-octet-Lewis-2D.png. License:
- Lewis carbon dioxide. Provided by: Wikimedia Commons. Located at: http://commons.wikimedia.org/wiki/File:Lewis_carbon_dioxide.gif. License:
- Acetic_acid_oxidation_state_analysis.png. Provided by: Wikimedia. Located at: https://commons.wikimedia.org/wiki/File:Acetic_acid_oxidation_state_analysis.png. License:
- 672px-Coordinate_Covalent_Bonding.svg.png. Provided by: Wikimedia. Located at: https://commons.wikimedia.org/wiki/File:Coordinate_Covalent_Bonding.svg. License: CC BY-SA: Attribution-ShareAlike
- Hypochlorite_Lewis_Structures_V1.svg. Provided by: Wikimedia. Located at: https://commons.wikimedia.org/wiki/File:Hypochlorite_Lewis_Structures_V1.svg. License: CC BY-SA: Attribution-ShareAlike
This chapter is an adaptation of the chapter “Lewis Dot Symbols and Lewis Structures” in Boundless Chemistry by LumenLearning and is licensed under a CC BY-SA 4.0 license.