71 Using Electrochemistry to Generate Electricity
LumenLearning
Dry Cell Battery
A dry-cell battery uses an immobilized electrolyte that minimizes moisture and allows for superior portability.
LEARNING OBJECTIVES
Discuss the operational components of a dry cell battery and their principal benefits
KEY TAKEAWAYS
Key Points
- A battery contains electrochemical cells that can store chemical energy to be converted to electrical energy.
- A dry-cell battery stores energy in an immobilized electrolyte paste, which minimizes the need for water.
- Common examples of dry-cell batteries include zinc-carbon batteries and alkaline batteries.
Key Terms
Defining a Dry Cell
In electricity, a battery is a device consisting of one or more electrochemical cells that convert stored chemical energy into electrical energy. The dry cell is one of many general types of electrochemical cells.
A dry cell has the electrolyte immobilized as a paste, with only enough moisture in it to allow current to flow. Unlike a wet cell, a dry cell can operate in any orientation without spilling, as it contains no free liquid. This versatility makes it suitable for portable equipment. By comparison, the first wet-cell batteries were typically fragile glass containers with lead rods hanging from an open top. They, therefore, needed careful handling to avoid spillage. The development of the dry-cell battery allowed for a major advance in battery safety and portability.
A common dry-cell battery is the zinc-carbon battery, which uses a cell that is sometimes called the Leclanché cell. The cell is made up of an outer zinc container, which acts as the anode. The cathode is a central carbon rod, surrounded by a mixture of carbon and manganese(IV) dioxide ([latex]\text{MnO}_2[/latex]). The electrolyte is a paste of ammonium chloride ([latex]\text{NH}_4\text{Cl}[/latex]). A fibrous fabric separates the two electrodes, and a brass pin in the center of the cell conducts electricity to the outside circuit.
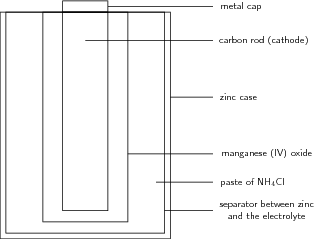
Chemical reactions occur in every part of the battery to allow for energy storage; the reactions can be described using balanced chemical equations that delineate the electron flow. The paste of ammonium chloride reacts according to the following half-reaction:
[latex]\text{2NH}_4 (aq) + \text{2e}^- \rightarrow \text{2NH}_3 (g) + \text{H}_2 (g)[/latex]
The manganese(IV) oxide in the cell removes the hydrogen produced by the ammonium chloride, according to the following reaction:
[latex]\text{2MnO}_2 (s) + \text{H}_2 (g) \rightarrow \text{Mn}_2\text{O}_3 (s) + \text{H}_2\text{O} (l)[/latex]
The combined result of these two reactions takes place at the cathode. Adding these two reactions together, we get:
[latex]\text{2NH}_4 (aq) + \text{2MnO}_2 (s) + \text{2e}^- \rightarrow \text{Mn}_2\text{O}_3 (s) + \text{2NH}_3 (g) + \text{H}_2\text{O} [/latex]
Finally, the anode half-reaction is as follows:
[latex]\text{Zn} (s) \rightarrow \text{Zn}^{2+} + \text{2e}^-[/latex]
Therefore, the overall equation for the cell is:
[latex]\text{Zn} (s) + \text{2MnO}_2 (s) + \text{2NH}_4 (aq) \rightarrow \text{Mn}_2\text{O}_3 (s) + \text{H}_2\text{O} (l) + \text{Zn}_2 + \text{2NH}_3 (g)[/latex]
The potential for the above reaction is 1.50 V.
Another example of a dry-cell battery is the alkaline battery. Alkaline batteries are almost the same as zinc-carbon batteries, except that the electrolyte used is potassium hydroxide ([latex]\text{KOH}[/latex]) rather than ammonium chloride. In some more modern types of so-called “high-power” batteries that have a much lower capacity than standard alkaline batteries, the ammonium chloride is replaced by zinc chloride.
Mercury Battery
Mercury batteries were a common electrochemical battery that were phased out of mainstream use in the U.S. by the 1996 Battery Act.
LEARNING OBJECTIVES
Discuss the applications of a mercury-oxide battery
KEY TAKEAWAYS
Key Points
- Mercury batteries were very common in the 20th century and were used in many common small and large appliances.
- Advantages of the mercury battery include its long shelf life and steady voltage output.
- Mercury batteries use a mercury compound as the cathode with a zinc anode.
- Along with other batteries that relied on heavy metals, mercury batteries were phased out by the Battery Act, which sought to decrease the environmental impact of disposable batteries.
Key Terms
A mercury battery, also called a mercuric oxide battery or a mercury cell, is a non-rechargeable electrochemical battery. These batteries have been used in the shape of button cells for watches, hearing aids, and calculators, and in larger forms for other devices, including walkie-talkies.
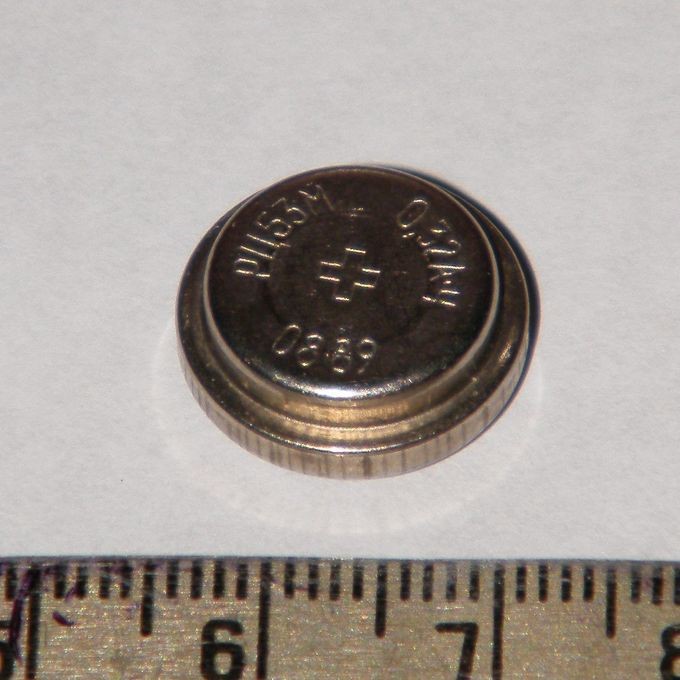
Mercury batteries have the advantages of a long shelf life of up to 10 years and steady voltage output. Although these batteries were very common in the mid-20th century, the Mercury-Containing and Rechargeable Battery Management Act (the Battery Act) passed in 1996 in the United States has largely phased out mercury batteries due to environmental concerns.
Mercury batteries use either pure mercuric oxide or a mixture of mercuric oxide with manganese dioxide as the cathode. Mercury oxide cells are constructed with a zinc anode, a mercury oxide cathode, and potassium hydroxide or sodium hydroxide as the electrolyte. Since mercuric oxide is a non-conductor, some graphite is mixed with it. This helps prevent the collection of mercury into large droplets. During discharge, zinc oxidizes to zinc oxide, and mercuric oxide gets reduced to elemental mercury. A little extra mercuric oxide is put into the cell to prevent evolution of hydrogen gas at the end of its life.
In a mercury battery, sodium hydroxide or potassium hydroxide is used as an electrolyte. Sodium hydroxide cells have nearly constant voltage at low discharge currents, making them ideal for hearing aids, calculators, and electronic watches. Potassium hydroxide cells, in turn, provide constant voltage at higher currents, making them suitable for applications requiring current surges, such as photographic cameras with flash and watches with a backlight. Potassium hydroxide cells also have better performance at lower temperatures.
The Battery Act
In 1996, the Mercury-Containing and Rechargeable Battery Management Act (the Battery Act; Public law 104-142) was signed into law in the United States. The intended objective of the act was to reduce heavy metals in municipal waste, streams, and ground water. This resulted from the disposal of mercury in single-use batteries, as well as of other toxic metal content such as lead from lead-acid batteries and the cadmium in rechargeable batteries. The law therefore sought to phase out the use of mercury in batteries due to the environmental damage it caused.
Lead Storage Battery
Lead-acid batteries provide high currents and store charge for long periods of time, making them essential for vehicles.
LEARNING OBJECTIVES
Recall the chemical reaction that occurs in lead storage batteries
KEY TAKEAWAYS
Key Points
- Lead- acid batteries, also known as lead storage batteries, can store a lot of charge and provide high current for short periods of time.
- The basic design of lead-acid batteries has not changed significantly since 1859 when Planté designed them, although some improvements were made by Faure.
- Lead-acid batteries are capable of being recharged, which is important for their use in cars.
- Discharging the stored energy relies on both the positive and negative plates becoming lead(II) sulfate and the electrolyte losing much of its dissolved sulfuric acid.
Key Terms
- lignosulfonate: Water-soluble anionic polyelectrolyte polymers; they are byproducts from the production of wood pulp using sulfite pulping.
Lead Batteries
A lead storage battery, also known as a lead-acid battery, is the oldest type of rechargeable battery and one of the most common energy storage devices. These batteries were invented in 1859 by French physicist Gaston Planté, and they are still used in a variety of applications. Most people are accustomed to using them in vehicles, where they have the ability to provide high currents for cranking power.
Although the batteries are reliable, they have a limited life, are heavy to ship, and contain toxic materials that require specific removal methods at the end of their useful life. Lead-acid batteries have moderate power density and good response time. Depending on the power conversion technology incorporated, batteries can go from accepting energy to supplying energy instantaneously. Lead-acid batteries are affected by temperature and must be maintained in order to achieve maximum life expectancy.
Designing a Lead Battery
In Planté’s design of the lead-acid cell, the positive and negative plates were made out of two spirals of lead foil, separated with a sheet of cloth, and coiled up. The cells initially had low capacity. A slow process of “forming” was required to corrode the lead foils, creating lead dioxide on the plates and roughening them to increase surface area. Planté plates are still used in some stationary applications, where the plates are mechanically grooved to increase surface area.
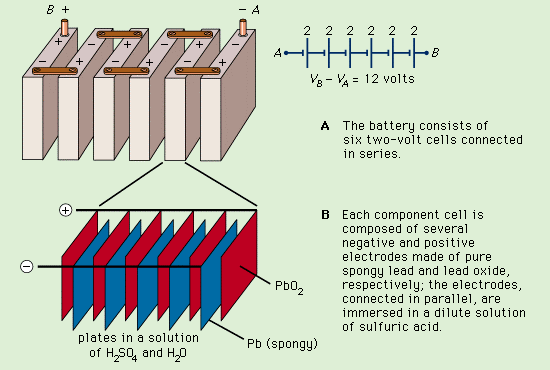
Camille Alphonse Faure’s pasted-plate construction is typical of automotive batteries today. Each plate consists of a rectangular lead grid. The holes of the grid are filled with a paste of red lead and 33 percent dilute sulfuric acid. This porous paste allows the acid to react with the lead inside the plate, which increases the surface area. Once dry, the plates are stacked with suitable separators and inserted into the battery container. An odd number of plates is usually used, with one more negative plate than positive. Each alternate plate is connected.
The paste contains carbon black, barium sulfate, and lignosulfonate. The barium sulfate acts as a seed crystal for the lead-to-lead sulfate reaction. The lignosulfonate prevents the negative plate from forming a solid mass during the discharge cycle, and instead enables the formation of long needle-like crystals. Carbon black counteracts the effect of inhibiting formation caused by the lignosulfonates.
Discharge Chemistry
In the discharged state, both the positive and negative plates become lead(II) sulfate (PbSO4). The electrolyte loses much of its dissolved sulfuric acid and becomes primarily water. The discharge process is driven by the conduction of electrons from the negative plate back into the cell at the positive plate in the external circuit.
Negative plate reaction: [latex]\text{Pb} (s) + \text{HSO}_4^- (aq) \rightarrow \text{PbSO}_4 (s) + \text{H}^+ (aq) + \text{2e}^-[/latex]
Positive plate reaction: [latex]\text{PbO}_2 (s) + \text{HSO}_4^- (aq) + \text{3H}^+ (aq) + \text{2e}^- \rightarrow \text{PbSO_4} (s) + \text{2H}_2\text{O}[/latex]
Combining these two reactions, one can determine the overall reaction:
[latex]\text{Pb} (s) + \text{PbO}_2 (s) + \text{2H}^+ (aq) + \text{2HSO}_4^- (aq) \rightarrow \text{2PbSO}_4 (s) + \text{2H}_2\text{O} (l)[/latex]
Charge Chemistry
This type of battery can be recharged. In the charged state, each cell contains negative plates of elemental lead ([latex]\text{Pb}[/latex]) and positive plates of lead(IV) oxide ([latex]\text{PbO}_2[/latex]) in an electrolyte of approximately 4.2 M sulfuric acid ([latex]\text{H}_2\text{SO}_4[/latex]). The charging process is driven by the forcible removal of electrons from the positive plate and the forcible introduction of them to the negative plate by the charging source.
Negative plate reaction: [latex]\text{PbSO}_4 (s) + \text{H}^+ (aq) + \text{2e}^- \rightarrow \text{Pb} (s) + \text{HSO}_4^- (aq)[/latex]
Positive plate reaction: [latex]\text{PbSO}_4 (s) + \text{2H}_2\text{O} (l) \rightarrow \text{PbO}_2 (s) + \text{HSO}_4^- (aq) + \text{3H}^+ (aq) + \text{2e}^-[/latex]
Combining these two reactions, the overall reaction is the reverse of the discharge reaction:
[latex]\text{2PbSO}_4 (s) + \text{2H}_2\text{O} (l) \rightarrow \text{Pb} (s) + \text{PbO}_2 (s) + \text{2H}^+ (aq) + \text{2HSO}_4^- (aq)[/latex]
Notice how the charging reaction is the exact opposite of the discharge reaction.
Other Rechargeable Batteries
The demand for many varieties of rechargeable batteries is due to their lower cost and lower environmental impact.
LEARNING OBJECTIVES
Discuss the general characteristics of rechargeable batteries
KEY TAKEAWAYS
Key Points
- Rechargeable batteries store energy through a reversible chemical reaction, which allows charge to be stored again after the battery has been drained.
- Rechargeable batteries have lower total cost of use and environmental impact than disposable batteries, which might be why the U.S. demand for rechargeable batteries is growing much faster than is the demand for non-rechargeable batteries.
- Common types of rechargeable batteries are lead-acid, nickel-cadmium ([latex]\text{NiCd}[/latex]), nickel- metal hydride ([latex]\text{NiMH}[/latex]), lithium- ion ([latex]\text{Li-ion}[/latex]), lithium-ion polymer ([latex]\text{LiPo}[/latex]), and rechargeable alkaline batteries.
Key Terms
- secondary cell: An electric cell that is rechargeable because it converts chemical energy into electrical energy by a reversible chemical reaction.
- energy density: The amount of energy that can be stored relative to the volume of the battery.
Rechargeable Batteries
A rechargeable battery is a type of electrical battery that is comprised of one or more electrochemical cells. It is known as a secondary cell because its electrochemical reactions are electrically reversible. In other words, after the stored charge has been drained, the battery’s chemical reactions can occur again, in reverse, to store a new charge. The U.S. demand for rechargeable batteries is growing twice as fast as the demand for non-rechargeable batteries, in part because rechargeable batteries have lower environmental impact and total cost of use than do disposable batteries.
Grid energy storage applications use rechargeable batteries for load-leveling. Load-leveling involves storing electric energy for use during peak load period. By charging batteries during periods of low electrical demand for use during periods of high demand, load-leveling helps eliminate the need for expensive peaking power plants and helps reduce the cost of generators over more hours of operation.
Rechargeable Battery Construction
As with all batteries, rechargeable batteries consist of an anode, a cathode, and an electrolyte. During charging, the anode material is oxidized, producing electrons, and the cathode is reduced, consuming electrons.
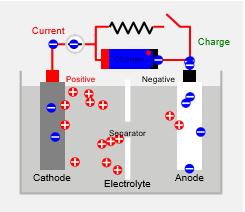
These electrons constitute the current flow in the external circuit. The electrolyte may serve as a simple buffer for internal ion flow between the electrodes, as in lithium-ion and nickel-cadmium cells, or it may be an active participant in the electrochemical reaction, as in lead-acid cells.
Types of Rechargeable Batteries
Several different combinations of chemicals are commonly used in rechargeable batteries. Different types include lead-acid, nickel-cadmium ([latex]\text{NiCd}[/latex]), nickel-metal hydride ([latex]\text{NiMH}[/latex]), lithium-ion ([latex]\text{Li-ion}[/latex]), lithium-ion polymer ([latex]\text{LiPo}[/latex]), and rechargeable alkaline batteries.
Lead-Acid Batteries
Lead-acid batteries, invented in 1859 by French physicist Gaston Planté, are the oldest type of rechargeable battery. Their ability to supply high-surge currents means that the cells maintain a relatively large power-to-weight ratio. These features, along with their low cost, make them attractive for use in motor vehicles, which require high currents.
Nickel-Metal Hydride Batteries
A nickel-metal hydride battery, abbreviated [latex]\text{NiMH}[/latex] or [latex]\text{Ni-MH}[/latex], is very similar to the nickel-cadmium cell ([latex]\text{NiCd}[/latex]). [latex]\text{NiMH}[/latex] batteries use positive electrodes of nickel oxyhydroxide ([latex]\text{NiOOH}[/latex]), as does the [latex]\text{NiCd}[/latex], but the negative electrodes use a hydrogen-absorbing alloy instead of cadmium. A [latex]\text{NiMH}[/latex] battery can have two to three times the capacity of a [latex]\text{NiCd}[/latex] battery of equivalent size, and its energy density approaches that of a lithium-ion cell.
Lithium-Ion Batteries
The lithium-ion battery is a family of rechargeable batteries in which lithium ions move from the negative electrode to the positive electrode during discharge, and back when charging. The negative electrode of a conventional lithium-ion cell is made from carbon. The positive electrode is a metal oxide, and the electrolyte is a lithium salt in an organic solvent. They are one of the most popular types of rechargeable battery for portable electronics, with one of the best energy densities and only a slow loss of charge when not in use. Lithium ion batteries are more expensive than [latex]\text{NiCd}[/latex] batteries but operate over a wider temperature range while being smaller and lighter. They are fragile and thus need a protective circuit to limit peak voltages.
Lithium-Ion Polymer Batteries
Lithium-ion polymer ([latex]\text{LiPo}[/latex]) batteries are usually composed of several identical secondary cells in parallel to increase the discharge-current capability. They are often available in series “packs” to increase the total available voltage. Their primary distinction from lithium-ion batteries is that their lithium salt electrolyte is not held in an organic solvent. Instead, it is in a solid polymer composite, such as polyethylene oxide or polyacrylonitrile. The advantages of [latex]\text{LiPo}[/latex] over the lithium-ion design include potentially lower cost of manufacture, adaptability to a wide variety of packaging shapes, reliability, and ruggedness. Their major disadvantage is that they hold less charge.
Alkaline Batteries
There are also rechargeable forms of alkaline batteries, which are a type of primary battery dependent upon the reaction between zinc ([latex]\text{Zn}[/latex]) and manganese dioxide ([latex]\text{MnO}_2[/latex]). They are manufactured fully charged and have the ability to carry their charge for years, longer than most [latex]\text{NiCd}[/latex] and [latex]\text{NiMH}[/latex] batteries, which self-discharge. Rechargeable alkaline batteries can also have a high recharging efficiency and have less environmental impact than disposable cells.
The Lithium-Ion Battery
Lithium-ion batteries are rechargeable batteries commonly used in consumer electronics; they rely on [latex]\text{Li}^+[/latex] migration.
LEARNING OBJECTIVES
Discuss the chemical transformations that occur in a lithium-ion battery during charge and discharge
KEY TAKEAWAYS
Key Points
- Superior energy densities, no memory effect, and only a slow loss of charge when not in use make lithium- ion batteries common for use in consumer electronics, military, electric vehicle, and aerospace applications.
- The anode is typically a lithium-containing compound and the cathode is typically a carbon-containing compound.
- The discharging reaction relies on the lithium ion from the electrolyte being extracted from the cathode and moved to the anode, while the opposite is true in the charging reaction.
Key Terms
Lithium-ion batteries (Li-ion batteries, or LIBs) are a family of rechargeable batteries in which lithium ions move from the negative electrode to the positive electrode during discharge. The ions follow the reverse path when the battery is charging. Li-ion batteries use a lithium compound as the electrode material.
Uses for Lithium-Ion Batteries
Lithium-ion batteries are common in consumer electronics. They are one of the most popular types of rechargeable battery for portable electronics because they have one of the best energy densities and only a slow loss of charge when not in use.
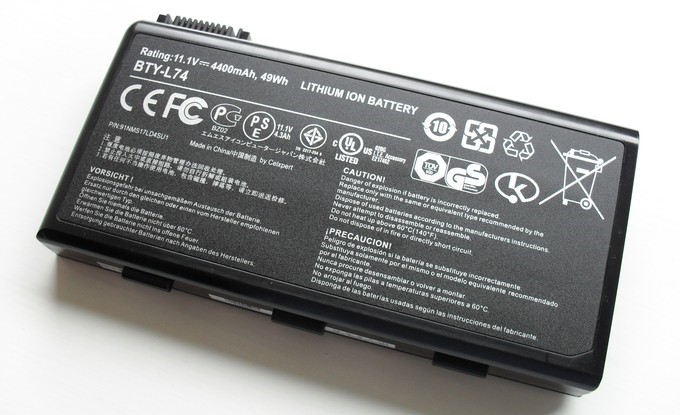
Beyond the field of consumer electronics, LIBs are also growing in popularity for military, electric vehicle, and aerospace applications. Research is yielding a stream of improvements to traditional LIB technology, focusing on energy density, durability, cost, and safety.
Types of Lithium-Ion Batteries
Chemistry, performance, cost, and safety characteristics vary with the type of LIB. Handheld electronics mostly use LIBs based on lithium cobalt oxide ([latex]\text{LCO}[/latex]), which offer high energy density but have well-known safety concerns, especially when damaged. Lithium iron phosphate ([latex]\text{LFP}[/latex]), lithium manganese oxide ([latex]\text{LMO}[/latex]), and lithium nickel manganese cobalt oxide ([latex]\text{LiNMC}[/latex]) batteries offer lower energy density but longer lives and inherent safety. These chemical compositions, or chemistries, are being widely used for powering electric tools and medical equipment.
Charging and Discharging
The three participants in the electrochemical reactions in a lithium-ion battery are the anode, the cathode, and the electrolyte. Both the anode, which is a lithium-containing compound, and the cathode, which is a carbon-containing compound, are materials into which lithium ions can migrate. The electrolyte is a lithium salt in an organic solvent. When a lithium-based cell is discharging, the positive lithium ion is extracted from the cathode and inserted into the anode, releasing stored energy in the process. When the cell is charging, the reverse occurs.
Materials for Cathodes and Anodes
The most commercially popular cathode material is graphite. The anode is generally one of three materials: a layered oxide (such as lithium cobalt oxide), a polyanion (such as lithium iron phosphate), or a spinel (such as lithium manganese oxide). The electrolyte is typically a mixture of organic carbonates, such as ethylene carbonate or diethyl carbonate, containing complexes of lithium ions.
In a lithium-ion battery, the lithium ions are transported to and from the cathode or anode. The transition metal, cobalt ([latex]\text{Co}[/latex]), oxidizes from [latex]\text{Co}^{3+}[/latex] to [latex]\text{Co}^{4+}[/latex] during charging and reduces from [latex]\text{Co}^{4+}[/latex] to [latex]\text{Co}^{3+}[/latex] during discharge.
Fuel Cells
Fuel cells are a compelling alternative to batteries, but they are still in the early stages of development.
LEARNING OBJECTIVES
Discuss the operation of a typical fuel cell
KEY TAKEAWAYS
Key Points
- A fuel cell is a device that converts the chemical energy from fuel into electricity via a chemical reaction with oxygen or another oxidizing agent.
- Batteries work in a closed system, while fuel cells require their reactants to be replenished.
- Using hydrogen as the major fuel source in fuel cells has several pros and cons that have kept it controversial for mainstream use.
- Fuel cells are made up of three adjacent segments: the anode, the electrolyte, and the cathode.
Key Terms
- anode: The electrode of an electrochemical cell at which oxidation occurs.
- fuel cell: A device that converts the chemical energy from a fuel into electricity through a chemical reaction with oxygen or another oxidizing agent.
- cathode: The electrode of an electrochemical cell at which reduction occurs.
- battery: A device that produces electricity by a chemical reaction between two substances.
Introduction and History
A fuel cell is a device that converts the chemical energy from fuel into electricity via a chemical reaction with oxygen or another oxidizing agent. Hydrogen is the most common fuel, but hydrocarbons such as natural gas and alcohols are sometimes used. Fuel cells are different from batteries in that they require a constant source of fuel and oxygen to run, but they can produce electricity continually for as long as these inputs are supplied. The development of miniature fuel cells can provide a cheap, efficient, and reusable alternative to batteries.
William Grove developed the first crude fuel cells in 1839. The first commercial use of fuel cells was in NASA space programs to generate power for probes, satellites, and space capsules. Currently, fuel cells are used for primary and backup power for commercial, industrial, and residential buildings and in remote or inaccessible areas. They are used to power fuel-cell vehicles, including automobiles, buses, forklifts, airplanes, boats, motorcycles, and submarines.
Fuel Cell Structure and Function
There are many types of fuel cells, but they all consist of an anode, which is the negative side, a cathode, which is the positive side, and an electrolyte, which allows charges to move between the two sides of the fuel cell.
Electrons are drawn from the anode to the cathode through an external circuit, producing direct-current electricity. Fuel cells are classified by the electrolyte they use, which is the main difference among the various types of fuel cells. Individual fuel cells produce relatively small electrical potentials, about 0.7 volts, so cells are “stacked,” or placed in series, to increase the voltage. In addition to electricity, fuel cells produce water, heat, and, depending on the fuel source, very small amounts of nitrogen dioxide and other emissions. The energy efficiency of a fuel cell is generally between 40-60 percent; it can reach 85 percent if waste heat is captured for use.
Despite the variety of fuel cell types, they all work in the same general manner. Two chemical reactions occur at the interfaces of the three different segments. The net result of the two reactions is that fuel is consumed, water or carbon dioxide is produced, and an electric current is created, which can be used to power electrical devices, normally referred to as the “load.”
At the anode, a catalyst oxidizes the fuel, usually hydrogen, turning the fuel into a positively charged ion and a negatively charged electron. The electrolyte is a substance that is specifically designed so that ions can pass through it but electrons cannot. The freed electrons travel through a wire, creating the electric current. The ions travel through the electrolyte to the cathode. There, the ions are reunited with the electrons, and the two react with a third chemical, usually oxygen, to create water or carbon dioxide.
The Pros and Cons of Fuel Cells
The use of hydrogen fuel cells is controversial in some applications. First of all, since the energy used to produce the hydrogen is comparable to the energy in the hydrogen, it is inefficient, and therefore expensive. If conventional power plants were used to produce the hydrogen there would be, at best, no positive change in current pollution rates. Other types of fuel cells don’t face this problem. For example, biological fuel cells take glucose and methanol from food scraps and convert them into hydrogen and food for the bacteria that break it down.
There are several advantages to hydrogen fuel cells, though. If the electricity produced by clean, renewable energy sources, such as solar and wind power, is used to produce hydrogen, the energy can be stored more easily than in large battery complexes.
There are practical problems to be overcome as well. Although the use of fuel cells for consumer products is probable in the near future, most current designs won’t work if oriented upside-down. Additionally, current fuel cells cannot be scaled to the small size needed for portable devices such as cell phones. Current designs also require venting and therefore cannot operate under water. They may not be usable on aircraft because of the risk of fuel leaks through the vents. Finally, technologies for safe refueling of consumer fuel cells are not yet in place.
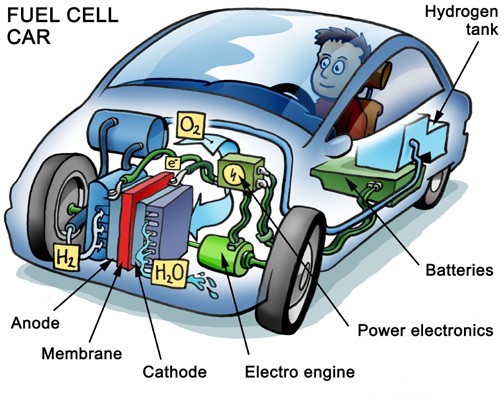
LICENSES AND ATTRIBUTIONS
CC LICENSED CONTENT, SHARED PREVIOUSLY
- Curation and Revision. Provided by: Boundless.com. License: CC BY-SA: Attribution-ShareAlike
CC LICENSED CONTENT, SPECIFIC ATTRIBUTION
- Free High School Science Texts Project, Siyavula Textbooks: Grade 12 Physical Science. September 17, 2013. Provided by: OpenStax CNX. Located at: http://cnx.org/content/m39485/1.1/?collection=col11244/latest. License: CC BY: Attribution
- Battery (electricity). Provided by: Wikipedia. Located at: http://en.wikipedia.org/wiki/Battery_(electricity). License: CC BY-SA: Attribution-ShareAlike
- Dry cell battery. Provided by: Wikipedia. Located at: http://en.wikipedia.org/wiki/Dry_cell_battery%23Dry_cell. License: CC BY-SA: Attribution-ShareAlike
- anode. Provided by: Wiktionary. Located at: http://en.wiktionary.org/wiki/anode. License: CC BY-SA: Attribution-ShareAlike
- cathode. Provided by: Wiktionary. Located at: http://en.wiktionary.org/wiki/cathode. License: CC BY-SA: Attribution-ShareAlike
- electrolyte. Provided by: Wiktionary. Located at: http://en.wiktionary.org/wiki/electrolyte. License: CC BY-SA: Attribution-ShareAlike
- Free High School Science Texts Project, Siyavula Textbooks: Grade 12 Physical Science. October 30, 2012. Provided by: OpenStax CNX. Located at: http://cnx.org/content/m39485/1.1/?collection=col11244/latest. License: CC BY: Attribution
- Batteries. Provided by: europe-versus-energy Wikispace. Located at: http://europe-versus-energy.wikispaces.com/Batteries. License: CC BY-SA: Attribution-ShareAlike
- Mercury-Containing and Rechargeable Battery Management Act. Provided by: Wikipedia. Located at: http://en.wikipedia.org/wiki/Mercury-Containing_and_Rechargeable_Battery_Management_Act. License: CC BY-SA: Attribution-ShareAlike
- Mercury battery. Provided by: Wikipedia. Located at: http://en.wikipedia.org/wiki/Mercury_battery. License: CC BY-SA: Attribution-ShareAlike
- electrolyte. Provided by: Wiktionary. Located at: http://en.wiktionary.org/wiki/electrolyte. License: CC BY-SA: Attribution-ShareAlike
- cathode. Provided by: Wiktionary. Located at: http://en.wiktionary.org/wiki/cathode. License: CC BY-SA: Attribution-ShareAlike
- anode. Provided by: Wiktionary. Located at: http://en.wiktionary.org/wiki/anode. License: CC BY-SA: Attribution-ShareAlike
- Free High School Science Texts Project, Siyavula Textbooks: Grade 12 Physical Science. October 30, 2012. Provided by: OpenStax CNX. Located at: http://cnx.org/content/m39485/1.1/?collection=col11244/latest. License: CC BY: Attribution
- Provided by: Wikipedia. Located at: http://en.wikipedia.org/wiki/File:??-53?.JPG. License: CC BY-SA: Attribution-ShareAlike
- Leadu2013acid battery. Provided by: Wikipedia. Located at: http://en.wikipedia.org/wiki/Lead%E2%80%93acid_battery. License: CC BY-SA: Attribution-ShareAlike
- Lead-acid batteries – Alaska Energy Wiki. Provided by: Wikidot. Located at: http://energy-alaska.wikidot.com/lead-acid-batteries. License: CC BY-SA: Attribution-ShareAlike
- chapter19. Provided by: np-apchemistry Wikispace. Located at: http://np-apchemistry.wikispaces.com/chapter19. License: CC BY-SA: Attribution-ShareAlike
- Leadu2013acid battery. Provided by: Wikipedia. Located at: http://en.wikipedia.org/wiki/Lead%E2%80%93acid_battery. License: CC BY-SA: Attribution-ShareAlike
- lignosulfonate. Provided by: Wikipedia. Located at: http://en.wikipedia.org/wiki/lignosulfonate. License: CC BY-SA: Attribution-ShareAlike
- Free High School Science Texts Project, Siyavula Textbooks: Grade 12 Physical Science. October 30, 2012. Provided by: OpenStax CNX. Located at: http://cnx.org/content/m39485/1.1/?collection=col11244/latest. License: CC BY: Attribution
- Provided by: Wikipedia. Located at: http://en.wikipedia.org/wiki/File:??-53?.JPG. License: CC BY-SA: Attribution-ShareAlike
- NP-APCHEMISTRY – chapter19. Provided by: Wikispaces. Located at: http://np-apchemistry.wikispaces.com/chapter19. License: CC BY-SA: Attribution-ShareAlike
- Alkaline battery. Provided by: Wikipedia. Located at: http://en.wikipedia.org/wiki/Alkaline_battery. License: CC BY-SA: Attribution-ShareAlike
- Lithium polymer battery. Provided by: Wikipedia. Located at: http://en.wikipedia.org/wiki/Lithium_polymer_battery. License: CC BY-SA: Attribution-ShareAlike
- Lead-acid battery. Provided by: Wikipedia. Located at: http://en.wikipedia.org/wiki/Lead-acid_battery. License: CC BY-SA: Attribution-ShareAlike
- Nickelu2013metal hydride battery. Provided by: Wikipedia. Located at: http://en.wikipedia.org/wiki/Nickel%E2%80%93metal_hydride_battery. License: CC BY-SA: Attribution-ShareAlike
- Rechargeable battery. Provided by: Wikipedia. Located at: http://en.wikipedia.org/wiki/Rechargeable_battery. License: CC BY-SA: Attribution-ShareAlike
- Rechargeable alkaline battery. Provided by: Wikipedia. Located at: http://en.wikipedia.org/wiki/Rechargeable_alkaline_battery. License: CC BY-SA: Attribution-ShareAlike
- Lithium-ion battery. Provided by: Wikipedia. Located at: http://en.wikipedia.org/wiki/Lithium-ion_battery. License: CC BY-SA: Attribution-ShareAlike
- Lead-acid battery. Provided by: Wikipedia. Located at: http://en.wikipedia.org/wiki/Lead-acid_battery. License: CC BY-SA: Attribution-ShareAlike
- Boundless. Provided by: Boundless Learning. Located at: http://www.boundless.com//chemistry/definition/energy-density. License: CC BY-SA: Attribution-ShareAlike
- Boundless. Provided by: Boundless Learning. Located at: http://www.boundless.com//chemistry/definition/secondary-cell. License: CC BY-SA: Attribution-ShareAlike
- Free High School Science Texts Project, Siyavula Textbooks: Grade 12 Physical Science. October 30, 2012. Provided by: OpenStax CNX. Located at: http://cnx.org/content/m39485/1.1/?collection=col11244/latest. License: CC BY: Attribution
- Provided by: Wikipedia. Located at: http://en.wikipedia.org/wiki/File:??-53?.JPG. License: CC BY-SA: Attribution-ShareAlike
- NP-APCHEMISTRY – chapter19. Provided by: Wikispaces. Located at: http://np-apchemistry.wikispaces.com/chapter19. License: CC BY-SA: Attribution-ShareAlike
- File:Secondary Cell Diagram.svg – Wikipedia, the free encyclopedia. Provided by: Wikipedia. Located at: http://en.wikipedia.org/w/index.php?title=File:Secondary_Cell_Diagram.svg&page=1. License: CC BY-SA: Attribution-ShareAlike
- Lithium-ion battery. Provided by: Wikipedia. Located at: http://en.wikipedia.org/wiki/Lithium-ion_battery. License: CC BY-SA: Attribution-ShareAlike
- electrolyte. Provided by: Wiktionary. Located at: http://en.wiktionary.org/wiki/electrolyte. License: CC BY-SA: Attribution-ShareAlike
- cathode. Provided by: Wiktionary. Located at: http://en.wiktionary.org/wiki/cathode. License: CC BY-SA: Attribution-ShareAlike
- anode. Provided by: Wiktionary. Located at: http://en.wiktionary.org/wiki/anode. License: CC BY-SA: Attribution-ShareAlike
- Free High School Science Texts Project, Siyavula Textbooks: Grade 12 Physical Science. October 30, 2012. Provided by: OpenStax CNX. Located at: http://cnx.org/content/m39485/1.1/?collection=col11244/latest. License: CC BY: Attribution
- Provided by: Wikipedia. Located at: http://en.wikipedia.org/wiki/File:??-53?.JPG. License: CC BY-SA: Attribution-ShareAlike
- NP-APCHEMISTRY – chapter19. Provided by: Wikispaces. Located at: http://np-apchemistry.wikispaces.com/chapter19. License: CC BY-SA: Attribution-ShareAlike
- File:Secondary Cell Diagram.svg – Wikipedia, the free encyclopedia. Provided by: Wikipedia. Located at: http://en.wikipedia.org/w/index.php?title=File:Secondary_Cell_Diagram.svg&page=1. License: CC BY-SA: Attribution-ShareAlike
- Li ion laptop battery. Provided by: Wikipedia. Located at: http://en.wikipedia.org/wiki/File:Li_ion_laptop_battery.jpg. License: CC BY-SA: Attribution-ShareAlike
- Miniature Fuel Cells – Thermal Systems. Provided by: Wikidot. Located at: http://me1065.wikidot.com/miniature-fuel-cells. License: CC BY-SA: Attribution-ShareAlike
- Electronics/Batteries. Provided by: Wikibooks. Located at: http://en.wikibooks.org/wiki/Electronics/Batteries%23Pros_and_cons_of_fuel_cells_in_various_applications. License: CC BY-SA: Attribution-ShareAlike
- Fuel cell. Provided by: Wikipedia. Located at: http://en.wikipedia.org/wiki/Fuel_cell. License: CC BY-SA: Attribution-ShareAlike
- fuel cell. Provided by: Wikipedia. Located at: http://en.wikipedia.org/wiki/fuel%20cell. License: CC BY-SA: Attribution-ShareAlike
- anode. Provided by: Wiktionary. Located at: http://en.wiktionary.org/wiki/anode. License: CC BY-SA: Attribution-ShareAlike
- cathode. Provided by: Wiktionary. Located at: http://en.wiktionary.org/wiki/cathode. License: CC BY-SA: Attribution-ShareAlike
- battery. Provided by: Wiktionary. Located at: http://en.wiktionary.org/wiki/battery. License: CC BY-SA: Attribution-ShareAlike
- Free High School Science Texts Project, Siyavula Textbooks: Grade 12 Physical Science. October 30, 2012. Provided by: OpenStax CNX. Located at: http://cnx.org/content/m39485/1.1/?collection=col11244/latest. License: CC BY: Attribution
- Provided by: Wikipedia. Located at: http://en.wikipedia.org/wiki/File:??-53?.JPG. License: CC BY-SA: Attribution-ShareAlike
- NP-APCHEMISTRY – chapter19. Provided by: Wikispaces. Located at: http://np-apchemistry.wikispaces.com/chapter19. License: CC BY-SA: Attribution-ShareAlike
- File:Secondary Cell Diagram.svg – Wikipedia, the free encyclopedia. Provided by: Wikipedia. Located at: http://en.wikipedia.org/w/index.php?title=File:Secondary_Cell_Diagram.svg&page=1. License: CC BY-SA: Attribution-ShareAlike
- Li ion laptop battery. Provided by: Wikipedia. Located at: http://en.wikipedia.org/wiki/File:Li_ion_laptop_battery.jpg. License: CC BY-SA: Attribution-ShareAlike
- Fuelcell. Provided by: Wikimedia Commons. Located at: http://en.wikipedia.org/wiki/Fuel_cell%23mediaviewer/File:Fuelcell.jpg. License: Public Domain: No Known Copyright
This chapter is an adaptation of the chapter “Batteries” in Boundless Chemistry by LumenLearning and is licensed under a CC BY-SA 4.0 license.
The electrode of an electrochemical cell at which reduction occurs.
The electrode of an electrochemical cell at which oxidation occurs.
Water-soluble anionic polyelectrolyte polymers; they are byproducts from the production of wood pulp using sulfite pulping.
battery designed to allow recharging
The amount of energy that can be stored relative to the volume of the battery.
devices similar to galvanic cells that require a continuous feed of redox reactants; also called a flow battery
single or series of galvanic cells designed for use as a source of electrical power