The Neuronal Membrane is Selectively Permeable
Objective 1: Explain which substances can cross the neuronal cell membrane by simple diffusion, and which need channel or pump proteins.
How Substances Cross the Neuronal Membrane (Permeability)
Gases can cross the membrane without assistance through simple diffusion. We’ll see a gas, nitric oxide (NO), that does this as it carries a neural signal.
Small, lipid-soluble molecules like steroids (modified cholesterol) can cross the membrane without assistance through simple diffusion. In this unit, we’ll see how neurosteroids do that. In Unit 14, we’ll see how the steroid hormones cross the cell membrane and act on intracellular receptors.
Water gets across the cell membrane without assistance, but that’s a miracle. No one has figured that one out yet.
Everything else needs some sort of carrier or channel to get across.
Ion Channels
In order to understand the working of nerve cells, we will focus on the movement of four ions:
- sodium (Na+)
- potassium (K+)
- calcium (Ca2+)
- chloride (Cl–)
In general, ion channels are selective. That means they prefer to pass just one ion on the above list. Some ion channels will pass more than one but they always have a preference for one ion over another.
We’ve already encountered the mechanically-gated ion channel as part of transduction in the somatosensory system, auditory system, and vestibular system. The mechanically-gated channel responds to pressure on the cell membrane by opening and letting sodium ions pass.
The remaining classes of ion channels that we haven’t discussed yet are shown in this diagram, and each will be discussed in detail.
- Leakage channels
- Voltage-gated channels
- Ligand-gated channels
- Signal-gated channels
Leakage Channels
The leakage channel is like a hole in a sieve that is specific for a certain ion. The most prominent kinds are potassium (K+) leakage channels and sodium (Na+) leakage channels. In a typical neuron, there are about a dozen times more potassium leakage channels than sodium leakage channels; we’ll see why this is important a bit later on. Even though they were named “leakage channel” because we thought they were always open, more recent evidence indicates their permeability can be regulated by intracellular messengers. Another name for this type of channel is an ungated channel to distinguish it from the gated channels we’ll look at later.
The concentration of potassium (K+) is almost always higher inside the neuron than outside. If we open a potassium channel, potassium goes from where it’s at higher concentration to where it’s at lower concentration: it goes from inside to outside the neuron.
The concentration of sodium (Na+) is almost always higher outside the neuron than inside. We’ve already seen, in Unit 3 and Unit 4 and in the clinic, that 0.9% (0.15 M) NaCl is a good blood substitute. If we open a sodium channel, sodium goes from where it’s at higher concentration to where it’s at lower concentration: it goes from outside to inside the neuron.
Voltage-Gated Channels
Now we turn our attention to the gated channels. These channels are either open or closed depending on conditions. If the channel is operated by a change in membrane voltage (membrane potential), we call it a voltage-gated channel. (Remember that voltage is a unit of electrical potential energy, so we will use these two words interchangeably throughout this unit.)
Voltage-gated channels are only found in a limited region of the neuron, typically on the surface of the axon, as shown by the yellow shading here.
The gating of these channels can be simple or complicated; we will study the simple gating of the voltage-gated potassium channel and the complicated gating of the voltage-gated sodium channel in Objective 4.
Ligand-gated channels
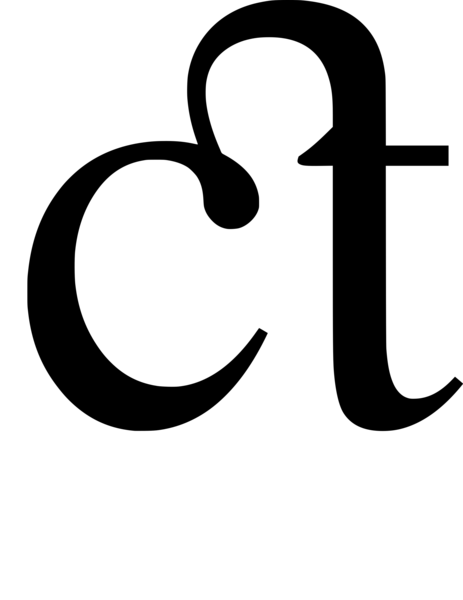
A ligand is any molecule that binds to a receptor. Usually we use the word ligand to mean either a naturally occurring neurotransmitter or a drug which either mimics the action of the neurotransmitter (an agonist) or a drug which blocks the action of the neurotransmitter (an antagonist).
The Latin word ligare means “to bind”, and we see it in the English words ligament, ligature, or ligate — all words that have to do with tying two things together, in this case a signaling molecule (the ligand) and a membrane-bound receptor. Ligand-gated channels are found wherever a signaling molecule can bind to a receptor and therefore changes the electrical properties of the neuron.
A closer look at a ligand-gated channel is shown at left. Here, we see a neurotransmitter molecule bound to a receptor. When the neurotransmitter is absent, the channel is closed. When the neurotransmitter (ligand) binds to the receptor, the shape of the receptor protein changes and causes the opening of an ion channel.
The opposite effect is also possible. Some neurotransmitters, when bound, cause an open channel to close.
In general, but certainly not always, these receptors are found on dendrites and cell bodies. The blue shading shows (in a general sense) the distribution of ligand-gated channels.
Signal-Gated Channels
The last type of ion channel we’ll consider is the signal-gated channel. Signal-gated channels change shape, and then open or close, depending on the presence of an intracellular signaling molecule. (Compare these to ligand-gated channels, that respond to an extracellular signaling molecule.)
These are usually operated, indirectly, by a G protein-coupled receptor (GPCR). They are also found at synapses, on the receiving side, so their distribution is the same as the ligand-gated channel (blue shading). We were introduced to the GPCR in Unit 4. We will study it in detail in Objective 12. The GPCR is also called a metabotropic (“metabolism-turned”) receptor because its activation causes a change in the biochemistry of the cell. This biochemical change results in an increase or decrease in the level of a specific intracellular signaling molecule, and that opens or closes an ion channel.
The distribution of neurotransmitter receptors is about the same, regardless of whether neurotransmitters bind to ligand-gated channels or G protein-coupled receptors. Because signal-gated channels respond to a nearby GPCR, the distribution of signal-gated ion channels is about the same as for ligand-gated ion channels.