The Eye and Retina
There are six extraocular muscles which are, as the name tells us, outside the eye. Working under the direction of a cortical area called the frontal eye fields, they guide the eyes to an exact, calculated target.
The six extraocular muscles occur in pairs. There is a lateral rectus and a medial rectus. These move each eye along a horizontal plane, from left to right.
There is a superior rectus and an inferior rectus. These muscles move each eye along a vertical plane, from up to down.
Finally, there are two oblique muscles which move the eye in more complicated ways that involve rotation and angular movement. These are the superior oblique and inferior oblique.
The cell bodies of lower motor neurons (α motor neurons) which innervate these muscles are found in three cranial nerve nuclei in the brainstem. The largest group forms cranial nerve III (the oculomotor nerve) which innervates the medial rectus, superior rectus, inferior rectus, and inferior oblique. The oculomotor nucleus makes up a large part of the midbrain tegmentum just anterior to the cerebral aqueduct.
The smallest group of neurons innervates the superior oblique muscle. Their axons form cranial nerve IV (the trochlear nerve), which exits the midbrain on the posterior (dorsal) side and wraps around the brainstem. This nerve is named after a bony loop in the eye socket called the trochlea (Latin: “pulley”). The tendon of the superior oblique runs through this loop, which redirects its action as shown in the diagram above.
There is a third group of neurons in the lower pons, near the junction of the pons and medulla. Their axons form the abducens nerve, which pulls the eye away from the midline. The Medical English term for this is “abduct”, so the activation of the abducens nerve abducts the eye.
The eye is divided into three confusingly-named regions: the anterior chamber (between the cornea and iris); the posterior chamber (surrounding the lens); and the vitreous chamber.
The most anterior part of the eye is a clear window called the cornea. The cornea is responsible for most of the refractory (focusing) power of the eye, as is clear from the use of contact lenses, or from its modification in the surgery called laser-assisted in situ keratomileusis (LASIK).
The iris also contains muscular tissue but its job is to change the size of the hole in the center of the iris — the pupil. Smooth muscle fibers which run around the rim of the pupil are called the pupillary sphincter and smooth muscle fibers which run radially in the iris are called the pupillary dilator. The main job of the iris is to shield the photoreceptors from an excessive number of light particle-waves (photons).
When there are very few photons around (i.e. in the dark), the pupillary sphincter relaxes and the pupillary dilator contracts, which increases the size of the pupil to its maximum size.
When there are a lot of photons around (i.e. in bright sunshine), the pupillary sphincter contracts and the pupillary dilator relaxes, reducing the size of the pupil to the smallest possible size and reducing the number of photons that reach the retinal photoreceptors.
A small pupil also helps the eye focus, one of the main reasons it’s easier to see objects more clearly in bright light than in dim light. A pinhole camera is able to focus an image without a lens, which is why it is used to visualize a solar eclipse. As is well known, staring directly at the sun in an eclipse can allow so many photons to flood into the eye, even through a small pupil, that damage to the photoreceptors results.
The lens and associated structures are found in the posterior chamber, the space lying between the iris and vitreous body.
The lens also participates in refraction, but unlike the cornea, its refraction is adjustable — at least early in life. A circular muscle called the ciliary muscle, part of the ciliary body, extends rope-like fibers called the suspensory ligaments which attach to the capsule surrounding the lens. When the eye focuses on a far object, the suspensory ligaments pull on the lens, flattening it.
When the eye focuses on a near object, the ciliary muscle relaxes, the suspensory ligaments go slack, and the natural elasticity of the lens pulls it into a rounder shape, allowing the eye to focus on near objects.
The problem occurs as we age and the natural elasticity of the lens decreases. It fails to round up as effectively as it had before, and we are stuck with the ability to only focus on faraway objects. This condition is a natural part of aging and is called presbyopia (literally, “elder vision”).
The shape of the eye is maintained by a jelly-like substance called the vitreous humor (“glassy liquid”).
The retina extends from the edges of the ciliary body, lining the interior of the eye.
In the direct path of the photons which pass through the pupil without being refracted (i.e. without the light rays being bent) is a pit called the fovea centralis (“central pit”). This region has a high concentration of yellowish visual pigment and so is also called the macula lutea (“yellow spot”).
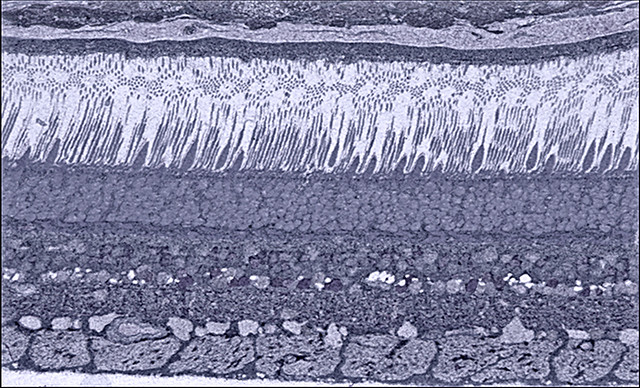
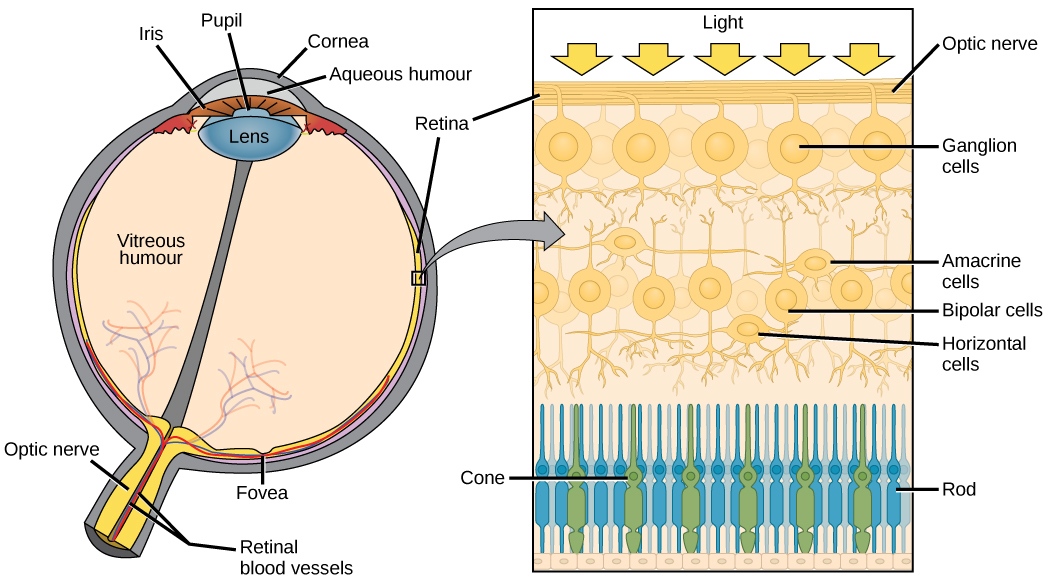
There are two types of photoreceptors, named after the distinctive shape of their modified cilia. In each case, the photoreceptor outer segments consist of stacks of membranes like a roll of pennies. The outer segment is connected to the cell body via a thin stalk.
Photons are particle-waves. This means they sometimes act as particles (such as when we talk about refraction of light rays by the cornea and lens) and sometimes act as waves (such as when we talk about their wavelength, or when we do an experiment called the two-slit experiment). The realization that light has this dual quality only came about at the turn of the 20th century and was truly a paradigm shift in our understanding of vision.
The different wavelengths of light possess different energy. This energy, which has both electrical and magnetic qualities, is accordingly called electromagnetic energy. An entire spectrum of electromagnetic energy exists, from the low-energy, long-wavelength radio waves at one end, to the high-energy, short-wavelength gamma rays at the other end. In between lie the familiar microwave energy, infrared energy, ultraviolet energy, and X-rays.
Tucked in between infrared and ultraviolet (as the names imply) is a relatively small slice of electromagnetic energies representing wavelengths from about 400 to about 700 nanometers (nm) or just a bit less than one thousandth of a millimeter. This is the visible spectrum, and it’s the series of wavelengths that can be detected by the human eye.
The longest wavelengths (lowest energies) in the visible spectrum are encoded by the visual system as red. The shortest wavelengths (highest energies) in the visible spectrum are encoded by the visual system as violet. In between lies an entire (literal) rainbow of colors: red, orange, yellow, blue, indigo, and violet. My engineer father taught me the mnemonic for this sequence by jokingly claiming the “inventor” of light was ROY G. BIV. I believed him.
If the membrane stack which extends from the photoreceptor cell body has the same diameter throughout, we call these cells rod photoreceptors. Rods are mostly indifferent to the wavelength of the incoming photons and are mostly interested in the number of photons. Incredibly, a rod photoreceptor can detect as few as 10 photons if the eye is perfectly adapted. This is the number of photons that enter the eye from a barely-visible distant star on a moonless night in the middle of the desert.
Rods are most sensitive to a single wavelength of light at about 500 nm (the actual number is a rather fussy 497.6 ± 3.3 nm) which appears to us as a gray-green color. As the wavelength increases above 500 nm or decreases below 500 nm, the rod photoreceptor becomes progressively less sensitive to light so it takes more photons to achieve the same electrochemical response. This is shown by the black curve.
If the membrane stack gets smaller as it gets further from the cell body, then we call this a cone photoreceptor. Cones are very sensitive to the wavelength of the incoming photon particle-waves. They code this information as color.
Cones come in three types. Long wavelength, or L cones, are sometimes called “red cones” though they respond best to a yellow-orange color at about 564 nm. Medium wavelength, or M cones, are sometimes called “green cones” and respond best to a yellow-green color at 534 nm. Short wavelength, or S cones, respond best to blue colors at 420 nm. (Note that the names are not the actual colors of the cones, but the colors that supposedly produce the “best” response.) Like the rods, each has a “favorite” color or wavelength. Photons with wavelengths near the “favorite” will cause a maximum response in that cone; as the wavelength moves away from the “favorite”, it takes more photons to produce the same response.
This setup allows the human retina to detect a complete range of colors in most cases. Each color in the visible spectrum gives us an unambigous “readout” from the three cones. For example, using the diagram, note that a wavelength of 520 nm (green light) reads near zero on the S cone, at 60% on the L cone, and at 80% on the M cone.
A wavelength of 630 nm (red light) reads near zero on the S cone, at 60% on the L cone, and at about 10% on the M cone.
A problem arises if either the L cone pigment or the M cone pigment are missing or altered, which happens in about 9% of males. Then it is easy to confuse the color red with the color green, because both produce the same readout on the L cone if the M cone pigment is missing, or the same readout on the M cone if the L cone pigment is missing. This condition is commonly called “color blindness” although people are not “blind” to these colors, they just confuse them. Because the genes for the M cone and L cone pigments are carried on the X chromosome, and women have two copies of this chromosome while men have only one, this relatively common mutation affects many more men than it does women.
Like some humans, dogs and other mammals such as deer have only two cone pigments (with absorbance maxima at 429 nm or blue and 555 nm at yellow-green) and are prone to confuse red and green. That’s why deer cannot easily distinguish between hunter orange and foliage green and why dogs cannot easily distinguish an orange training dummy in green grass.
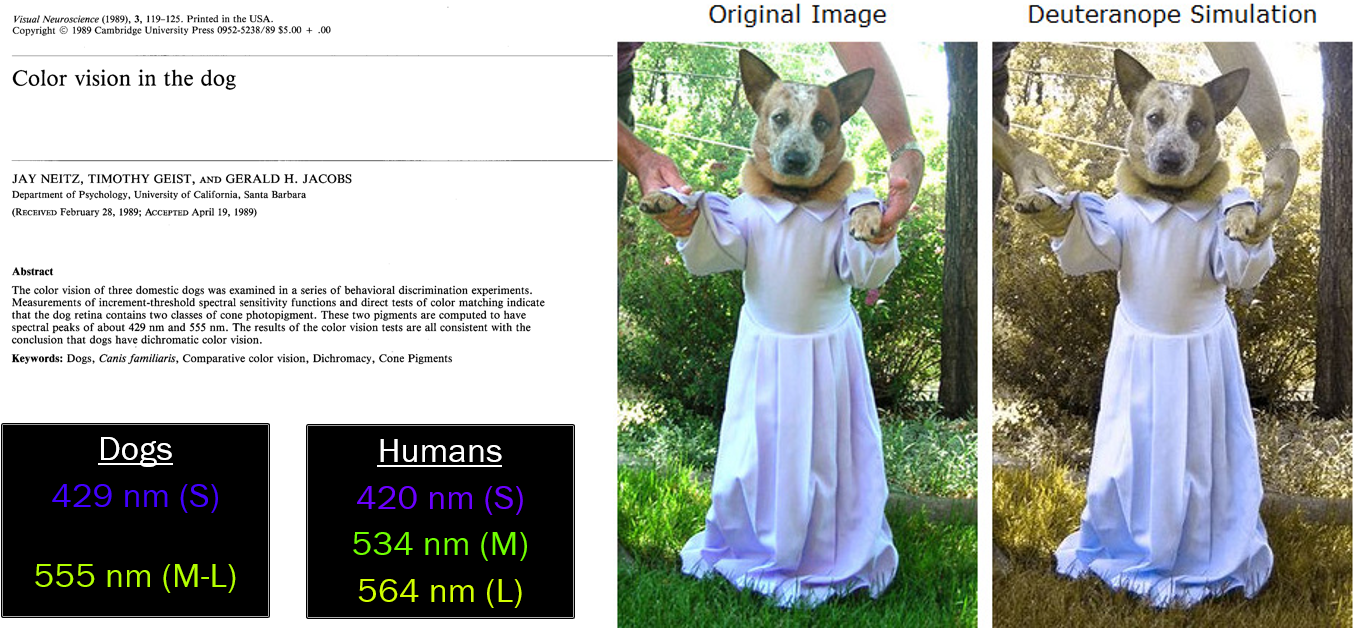
In the human retina, the other four cell types are “scraped away” at the foveal pit, leaving only the cone photoreceptors to detect the presence and wavelength of light. Thus the fovea (macula) is where visual acuity is the greatest. Almost all visual information comes from the central 5° of visual angle. (For comparison, the moon is about 0.5° of visual angle and the thumb at arm’s length is about 2° of visual angle, so the macula is about two and a half thumbs wide.) It takes a lot of motion, or a very large object, for us to detect visual stimuli outside of this central area. Without realizing it consciously, we move our eyes around to pick up elements of a visual scene in small ten-moon-diameter circles.
Just outside the macula, the number of cones decreases and rods predominate. This is why, to visualize a dim star, we need to look slightly away from it.

About 15° to the nasal side of the fovea is the blind spot, formed by the exiting ganglion cell axons that will form the optic nerve. Since no photoreceptors exist here, there is no perception of light from this region of the retina. Our visual system does a lot of work to make us unaware of the blind spot; contrariwise, we have to do a lot of work to be able to find our blind spot.