The Chemical Synapse
Objective 1: Label the key features of a chemical synapse.
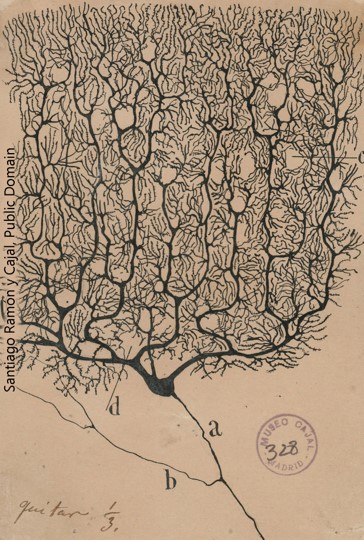
The synapse existed as a hypothesized, imaginary structure long before any neuroscientist ever saw one. In 1897, Sir Charles Sherrington coined the term “synapse” (from a Greek word meaning, “to clasp”) so that he could better explain his ideas about how nerve cells communicated with one another. Recall that histologists Camillo Golgi and Santiago Ramón y Cajal were battling over what seemed a black-or-white argument. Golgi believed that neurons form a syncytium, a continuous, electrically conductive network. On the other hand, Cajal claimed that neurons remained separate from one another, but his techniques did not allow him to see the contacts between nerve cells. Cajal’s beliefs, which became known as the Neuron Doctrine, dominated late 19th century neuroscience, and Sherrington was planting his physiological flag firmly in alliance with Cajal’s anatomical camp.
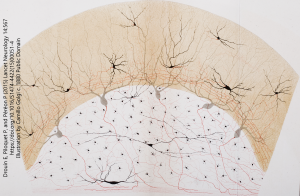
(Note that the words matter here: a “doctrine”, like the term “Central Dogma” used by molecular biologists, refers to a set of beliefs that may or may not be proven but are useful to organize and advance the research in the area. We now know that Golgi was a little bit right and Cajal was a little bit wrong, as we will see later when we discuss electrical synapses.)
Sherrington needed the concept of a synapse to explain how a nerve cell could influence the activity of a muscle cell. In the first half of the 20th century, physiologists and pharmacologists studied the nature of chemical signalling between nerve cells, and between nerve and muscle cells. In a critical and elegant 1920 experiment, Otto Loewi demonstrated the existence of a chemical message between the nerve endings of the vagus nerve and the heart muscle.
After dreaming the experimental design, Loewi manifested his dream: he put a frog heart with vagus nerve attached in one beaker, stimulated the vagus nerve, and observed that the heart rhythm slowed. This experiment had been done dozens of times by multiple investigators. His innovation, however, was to take a heart that had been denervated (the vagus nerve had been removed and was no longer attached to the heart) and then place the denervated heart in the saline solution that had previously housed the stimulated vagus-heart preparation. The second, denervated heart slowed down without direct contact with a vagus nerve. Loewi correctly assumed that a chemical messenger must have been released into the saline bath. Naturally enough, he named this messenger vagusstoff (“vagus stuff”). We now know that vagusstoff is a chemical called acetylcholine, which is released from the axonal terminals of the vagus nerve and binds to receptors found on the heart muscle.
Direct observation of the parts of a synapse had to wait until the invention of the electron microscope after the Second World War. Chemical messengers such as acetylcholine are stored in vesicles (Latin: “little bladders”) and are released when the region around the synapse is depolarized. A vesicle is about 40 nm in diameter, about 1/25 as big as the smallest object that can be seen under the light microscope. For vesicles full of acetylcholine at the neuromuscular junction, we can measure the number of neurotransmitter molecules in a single vesicle — what physiologists call a quantum (plural quanta). In 1954, del Castillo and Katz calculated the number of molecules in a quantum using statistical methods combined with clever pharmacology and physiology. There are about 10,000 acetylcholine molecules in a single synaptic vesicle.
The fusion of these vesicles with the neuronal cell membrane was shown directly by Heuser, Reese, and colleagues. In a 1979 paper, they described a device that would stimulate a nerve terminal as it hurtled towards a block of copper cooled to liquid helium temperatures (4K, or –269°C). Using this device, they observed vesicles fusing about 0.2 msec (i.e. 1/5000 of a second) after nerve stimulation.
The neuron that contains vesicles and releases them at the synapse is termed the presynaptic neuron. It is the neuron sending the information.
The neuron or muscle cell receiving the information is called the postsynaptic neuron. On its surface, specialized proteins called receptors bind to the signaling molecule. For example, at the neuromuscular junction between the vagus nerve axon terminals and the heart, acetylcholine binds to muscarinic acetylcholine receptors. Between a spinal nerve axon terminal and the skeletal muscle it innervates, acetylcholine binds to nicotinic acetylcholine receptors.
There is no good system for naming receptors. In many cases, the receptor is named by pharmacologists who studied the drug binding to a receptor, such as nicotine (from tobacco) binding to the nicotinic acetylcholine receptor, and muscarine (from a poisonous mushroom, Amanita muscaria) binding to the muscarinic acetylcholine receptor. In other cases, especially when the receptor was discovered using molecular biological techniques instead of pharmacological techniques, the receptors have various letter and number designations that follow gene naming conventions. An example of this is the HTR5A serotonin receptor.
No matter what they are named, receptors for water-soluble neurotransmitters, such as acetylcholine, gamma-aminobutyric acid (GABA), glutamate, glycine, dopamine, norepinephrine (noradrenaline), serotonin, or adenosine, are proteins embedded in the cell membrane. Two broad classes of receptors exist: those that allow ions to move across the cell membrane (ionotropic) and those that cause an internal change in the biochemistry of the receiving neuron (metabotropic).
Neurons also respond to steroid hormones (neurosteroids) such as the sex hormones 17-beta-estradiol and dihydrotestosterone. In those cases, since steroids can move across the cell membrane without help, the receptors are found in the cytoplasm and act to carry the steroids to the cell nucleus, where they have an effect on transcription of genes to make messenger RNA.
There are also neurotransmitters that are gases, most notably nitric oxide (chemical symbol NO). Nitric oxide and other gaseous neurotransmitters don’t have receptors, and they can travel in either direction. The gases, you might remember, pass easily through the cell membrane. Because of this, gases diffuse in all directions away from the enzyme that created them.
The invention of the electron microscope allowed cell biologists to see the synapse for the first time. This drawing is reproduced from a classic textbook of cell biology and electron microscopy, Don Fawcett’s The Cell. The vesicles were presumed to contain neurotransmitter, first based on electrophysiological evidence, and then later due to some clever combinations of electrophysiology and freeze-fracture electron microscopy.
Electron microscopists also saw an electron-dense black fuzz presynaptically, and sometimes postsynaptically. The nature of that fuzz was a mystery for some time. We now know that the presynaptic fuzz corresponds to the voltage-gated calcium channels and the complex protein machinery needed for fusion of vesicles with the cell membrane.
The postsynaptic fuzz represents the neurotransmitter receptors and the cytoskeletal scaffolding which holds those receptors in place.
This summary diagram shows all the parts of a canonical synapse, that is, one that you normally find in textbooks. Real synapses tend to deviate from this idealized representation in various ways but we have to start somewhere.
Elements typically found in a presynaptic terminal include:
- an enzyme or organelle to make the neurotransmitter;
- a mechanism for packaging the neurotransmitter into vesicles;
- voltage-gated calcium (Ca2+) channels which respond to a voltage change by opening and letting Ca2+ into the presynaptic terminal;
- a mechanism for responding to Ca2+ by fusing the vesicles to the presynaptic membrane, releasing the neurotransmitter into the synaptic cleft.
The last two of these constitute the active zone, a cluster of presynaptic machinery that can be seen using electron microscopy.
Elements typically found in a postsynaptic terminal include:
- receptors for turning binding of the transmitter into either an electrical change (ionotropic receptors) or a biochemical change (metabotropic receptors)
Ionotropic receptors are also termed ligand-gated channels. A ligand is anything that binds to a receptor; it can be the naturally occuring neurotransmitter, or a drug like nicotine or muscarine. When the ligand binds, the shape of the receptor protein changes, a gate opens, and specific ions are allowed to flow.
Metabotropic receptors are also termed G protein coupled receptors because they change the biochemistry (metabolism) of the cell by releasing an intracellular protein that binds to cyclic guanosine monophosphate (cGMP). When the receptor protein binds to a ligand, it changes shape and uncouples the G protein which then slides along the inner surface of the cell membrane to trigger a cascading series of biochemical reactions.
Some sort of mechanism for inactivating or removing the neurotransmitter are also an important part of the synapse. For example, acetylcholine is broken into its parts by an enzyme called acetylcholinesterase (it breaks the bond between acetate and choline at a chemical bond called an ester linkage). Peptide neurotransmitters, made up of strings of amino acids, are also inactivated in the same way; peptidases break the polypeptide chain into its individual amino acids. The resulting inactive parts are still needed by the presynaptic cell, and so carriers take these inactive parts back into the presynaptic cell for recycling.
Neurotransmitter is also removed from synapses by proteins called transporters. These are also termed reuptake mechanisms. For example, serotonin is taken up by serotonin transporters embedded in the presynaptic membrane. Drugs which block this process, like Celexa®, Lexapro®, or Prozac®, are classified as selective serotonin reuptake inhibitors for this reason. They block the transporter and leave more active serotonin available to bind to receptors over and over.