Organelles
Objective 5: List the organelles found in neurons and the basic function of each.
If you are reading this, then your body is made up of eukaryotic cells. The prefix eu– means “good, perfect, true”. The root karyon is a bit more problematic. The late 19th century histologists, looking through microscopes, needed to name the nucleus using Greek or Latin but no Athenian or Roman had a microscope and therefore had never seen a nucleus. So they went with the Greek word for “nut” meaning “in the center”, “surrounded by a shell”. Thus eukaryotic cells have a true nucleus.
The three parts of a eukaryotic cell are:
- the cell membrane
- the nucleus
- the cytoplasm
Parts of the cell are divided off from others, both structurally and functionally. These cell parts are called organelles (literally, “little organs”). Just like the organs of your body each have a specific job, the organelles of the cell have specific jobs to do and their structure reflects this.
The Neuronal Membrane
The cell membrane is also called the plasma membrane or the plasmalemma.
The cell membrane is formed from molecules called phospholipids, because they have both a water-loving head group and a water-hating pair of lipid tails.
The exact composition of both the head group and the tails may vary. Different head groups may associate differently with other cells or with the outside environment. The variations in the composition of tails allows the cell membrane to vary how stiff or loose it is, among other features. Cholesterol molecules (see section on steroid synthesis, below) are also wedged in between the tails to help regulate membrane fluidity.
Since the tail groups are made up of carbon and hydrogen, two atoms that share electrons equally in a non-polar covalent bond, they only associate with other lipid tails. The head groups consist of nitrogen, phosphorus, and oxygen molecules, all of which tend to share electrons unequally in a polar covalent bond. We use the terms non-polar/hydrophobic, and polar/hydrophilic, interchangeably to indicate this hatred of, or love for, water.
In this way the phospholipids form a lipid bilayer, with the tails grouped together and the heads sticking out into the watery environment outside and inside the cell.
The molecules that make up a cell membrane can shift slightly past one another, but they cannot flip. This constant molecular motion is an important feature of membranes. If we don’t want molecules moving around, we have to anchor them to the structural proteins of the cell, the cytoskeleton, which is discussed later.
Proteins are associated with the phospholipid bilayer, either floating like icebergs in the membrane or spanning the membrane to transfer signals from the outside to the inside or vice-versa. For example, ion channels form tunnels that allow only one or a few types of charged particles to cross the membrane.
The amino acids which make up protein can either associate freely with water or repel water. The portion of the protein which associates with the lipid tails tends to have the water-repelling, hydrophobic amino acids oriented toward the outside of the protein in the center of the lipid bilayer. In this way, the membrane-spanning proteins are held in place.
As shown here, when sugar molecules are attached to the protein, they form branched, tree-like structures that are found only on the outside of the cell. The sugar part of the molecule is called its glycan moiety, from the Greek word for “sweet” and the French word moité, meaning “half” or “portion”.
Nucleus
Let’s say you need to start a restaurant that only uses one-of-a-kind, heirloom recipes. You can’t afford to take the cookbooks and scraps of paper that contain the recipes into the kitchen — someone might spill tomato sauce on them, or worse, set one of them on fire and then it would be lost forever. Rather, you’d set up a system where the heirloom recipes were locked away for safekeeping, and only trained personnel could put on clean gloves, copy the recipes, and return the original to its storage place.
That’s what goes on in the nucleus. There are two main functions of the deoxyribonucleic acid (DNA) in the nucleus. One is to be copied, then each copy is packed into shipping boxes to be divided between the old restaurant location and the new startup. This copying is called DNA replication. We’ll discuss this a bit more later on, but DNA is duplicated during the S (synthesis) phase of the cell cycle. Very few neurons go through this process after the brain is fully formed (e.g. after birth in humans). When the DNA is duplicated, it is packaged at the beginning of the M (mitosis, from the Greek word for “thread-process”) phase of the cell cycle. Threads of DNA coalesce into chromosomes (Greek, “colored bodies“).
It’s important not to underestimate the breathtakingly complex process that packages DNA. The largest DNA molecule in the human, chromosome 1, is 247 million DNA base pairs. All the DNA molecules in a single cell, laid end-to-end would span about 3 meters. That 3 meters needs to be packed into a nucleus that is less than 1/100,000 of a meter in diameter. The sugar-phosphate backbone of DNA is studded with negative charges; the negatively-charged DNA has to be wrapped around a positively-charged histone protein in order to achieve this packing density.
But in order to be read, the DNA “recipe” has to be unspooled so ribonucleic acid (RNA) polymerase can act on it. The ending –ase indicates an enzyme; a polymer is complex structure made up of repeating subunits. Therefore, RNA polymerase is an enzyme that joins RNA subunits into a long RNA molecule, called messenger RNA (mRNA).
The mRNA is pulled out of the nucleus through a protein structure called a nuclear pore. Then the mRNA can serve as a template (“recipe”) for protein synthesis. Ribosomes are a macromolecular machine that turns nucleic acid into protein. Ribosomes are either found “free” (alone) in the cytoplasm or may be associated with membranes in an organelle called the rough endoplasmic reticulum (rER).
Rough Endoplasmic Reticulum (RER or rER)
Ribosomes, and the system of membranes they’re associated with, are collectively called the rough endoplasmic reticulum (RER or rER). The word reticulum means a “little network”; endoplasm means this organelle is found in the cytoplasm of the cell.
Free ribosomes, not associated with a membrane, are generally believed to make proteins that are needed inside the cell. On the other hand, the rER makes proteins that are either found in vesicles that are secreted from the cell, or on the cell surface. For example, peptide neurotransmitters such as the endogenous opioids, made up of strings of amino acids, are made in the rER. Cell surface receptors, like the ligand-gated channels we’ll see later, are also made in the rER. Any protein, which is a string (polymer) of amino acids, is made by ribosomes.
Since nucleic acids avidly take up cresyl violet and other aniline dyes, they stain dark purple in conventional sectioning and staining methods. The rER of neurons (only visible in the electron microscope) is collectively called the Nissl substance when viewed through the light microscope.
Golgi Complex (Golgi Apparatus, Golgi Body)
Amino acids are joined together in a long polymer to form polypeptides or proteins. In general, these proteins must be further processed, either by removing parts or adding parts or refolding them into different shapes than how they were made.
Here in the Golgi complex, a number of different chemical groupings may be added to proteins, for example:
- sugar groups (glycosylation)
- acetyl groups (acetylation)
- phosphate groups (phosphorylation)
- methyl groups (methylation)
- sulfate groups (sulfation)
- small ubiquitin-related modifier (SUMO) groups (SUMOylation)
- farnesyl groups (farnesylation)
- geranylgeranyl groups (geranylgeranylation)
These chemical alterations are collectively called post-translational modifications because they occur after the mRNA is translated into protein by the ribosome.
All of these processes occur in different locations within the Golgi. That is, different areas of the Golgi are specialized for different functions. As everyone knows, you don’t want to farnesylate where you geranylgeranylate.
Newly-formed polypeptide or protein chains enter the Golgi at the forming face (also called the cis face, because it is closest to the rER) and fully processed proteins ready for export are packaged into vesicles at the leaving face (also called the trans face, because it is furthest from the rER).
The Endomembrane System
Transport vesicles bud off the leaving face of the Golgi. Vesicles (Latin: “little bladders”) are small, round, membrane-bound bags that have three possible fates:
- they can travel to the cell membrane, fuse, and release their contents (exocytosis);
- they can travel to the cell membrane, fuse, and display proteins or glycoproteins on the surface;
- they can fuse with lysosomes and their contents are destroyed.
Neurons are really good at the first of these, especially neurons that release neurotransmitter signaling molecules at synapses. We will study exocytosis in more detail in a later chapter.
The second process is used to express cell surface receptors, such as neurotransmitter receptors, on the neuronal cell membrane.
The third process is used to recycle proteins that are mis-folded, malformed, or otherwise unusable; in this way the valuable amino acids that make up the protein are recycled.
In this way, the nucleus, rER, Golgi, and vesicles form a single, continuous assembly line called the endomembrane system.
Smooth Endoplasmic Reticulum: Garbage Disposal
Electron microscopists discovered several different types of membrane networks inside the cell. It was easy to distinguish those that had ribosomes (rough endoplasmic reticulum) from those that didn’t (smooth endoplasmic reticulum). But we now know that smooth endoplasmic reticulum can be divided into at least three different subtypes, which are not distinguishable with conventional electron microscopic techniques but have completely different functional roles.
In cells that are responsible for breaking down unwanted or toxic molecules, especially those that are lipid-soluble, there is an extensive network of smooth ER which is contains the chemical constituents so breakdown can take place.
The liver detoxifies a number of substances, breaking them down with the cytochrome P450 family of enzymes which are bound to these membranes. For example, ethanol may be broken down in the microsomal ethanol oxidizing system.
Lysosomes
Lysosomes are membrane-bound vesicles that have a very acidic solution inside. The lysosomal contents would be damaging to the cytoplasm of the cell.
If, on the other hand, your intent is to break a molecule down, then dumping into a lysosome is an excellent choice. Between the acidic environment (pH 5, about 100X more acidic than the cytoplasm) and the acid-loving enzymes that are found inside of lysosomes, they provide an ideal environment to break chemical bonds, whether the peptide bonds that hold amino acids together in a protein or the non-polar covalent bonds that hold carbons and hydrogens together in a lipid molecule.
The enzymes found inside of lysosomes are called, as a group, acid hydrolases. They work best inside the acid environment of the lysosome, accounting for the first part of the name; and they catalyze hydrolysis reactions, accounting for the second part of the name. (The ending –ase tells us it’s an enzyme.) Hydrolysis reactions use a water molecule to break apart a chemical bond. There are 40 different kinds of acid hydrolases found in the lysosome, including those that break down nucleic acids (nucleases); those that break down proteins (proteases); those that break down lipids and phospholipids (lipases and phospholipases).
The acid environment of the lysosome is maintained by a proton (H+) pump. This protein sits astride the vesicular membrane and moves hydrogen ions into the vesicle using ATP as an energy source. As we’ll see later, proton pumps are a common feature of both lysosomes and neurotransmitter-containing vesicles.
Mitochondria
Mitochondria have an interesting backstory. About 1.45 billion years ago, a bacterial cell was jealous of its neighbor who was so much more efficient at making adenosine triphosphate (ATP), the energy currency of the cell. So in a massive show of bacterial force, the energy-poor grasshopper cell swallowed the industrious ant cell and enslaved it. (The technical, but much less dramatic, term for this process is endosymbiosis).
The cytoplasm contains the enzymes needed for glycolysis, the breakdown of glucose molecules to form a net 2 ATP molecules per glucose. The end-product of glycolysis is a 3-carbon sugar called pyruvate.
Without mitochondria, or the oxygen which make mitochondria operate, pyruvate production is a dead end, resulting in the buildup of lactic acid. (But the brain, unlike other tissues, appears to make use of lactic acid; see the later chapter on this.)
With oxygen, mitochondria take up pyruvate and oxygen and carry them through a series of chemical and even atom-splitting reactions to form the end products carbon dioxide (CO2), water, and two to three dozen ATP molecules per glucose molecule. This tremendous efficiency in ATP production is what the ancient bacterium was so jealous about. Accordingly, mitochondria have a whole crapton of different proteins (that we will study later) including the components of the citric acid cycle and the electron transport chain, all of which are incredibly efficient ATP producers.
All these proteins need room, so the surface area of the inside of mitochondria is enhanced by cristae, which give the mitochondrion its characteristic wrinkled appearance.
Smooth Endoplasmic Reticulum and Mitochondria: Steroid Synthesis
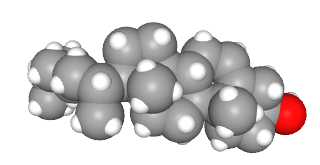
Cholesterol is important as a structural molecule. It is a significant constituent of cell membranes, where it regulates membrane fluidity (i.e., the ability of molecules to move around inside of membranes). Membrane fluidity, on the other hand, is especially important for cells placed in extreme environments. In general, cell membranes belonging to animals in the Antarctic climate have short fatty acid chains and very little cholesterol where cell membranes belonging to desert creatures may have longer fatty acid chains and a lot more cholesterol.
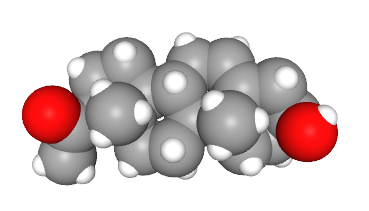
Cholesterol also is used to make neurotransmitters and hormones in both the nervous system and in the endocrine system, respectively.
Nervous system chemical signaling molecules made from cholesterol are called neurosteroids.
Neurosteroids are made by another cytochrome P450 enzyme system found in mitochondria. Mitochondria and smooth endoplasmic reticulum are the sites of steroid synthesis in neurons and other cells.
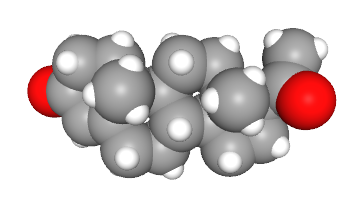
For example, the molecule progesterone is used both in the nervous system as a neurosteroid and in the endocrine system as a hormone during the estrus cycle in most animals and the menstrual cycle in humans.
Neurosteroid synthesis occurs in mitochondria and in the smooth endoplasmic reticulum. These mitochondria are slightly different in morphology. Mitochondria engaged in energy metabolism have cristae which appear more rectangular in cross-section while mitochondria engaged in steroid synthesis have cristae that are more tubular in cross-section.
This electron micrograph is of a non-neuronal cell called a Leydig (interstitial) cell. Its job is to respond to the extracellular signal luteinizing hormone and make the neurosteroid (but also characteristically male) signaling molecule testosterone. There are multiple pathways for testosterone synthesis, but one of them is from cholesterol to pregnenolone to progesterone (as shown above), then from progesterone to androstenedione to testosterone (as shown here).
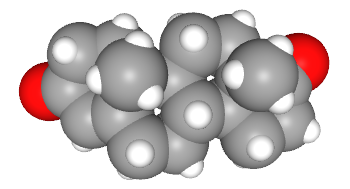
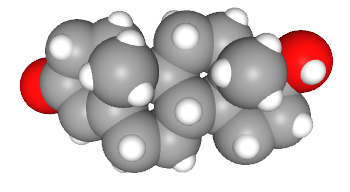
The Cytoskeleton
There are three components to the cytoskeleton:
- actin filaments, also called microfilaments
- intermediate filaments, made up of many different proteins
- microtubules, made up of α-tubulin, β-tubulin, and a group of proteins collectively called microtubule-associated proteins (MAPs)
The actin protein exists in two forms: globular actin (G-actin), which is an individual actin molecule; and filamentous actin (F-actin), which is a string of globular actin molecules joined together in a chain.
This drawing, modified from a pen and ink original by Keith Porter (who trained Jim Hutchins), shows the complicated geometry of the cytoskeleton as seen through the high voltage electron microscope.
In the past 50 years since Fawcett made his pen-and-ink drawings, we’ve advanced microscopy to the point where we can visualize the cytoskeleton directly using fluorescence microscopy, as seen here.
These structural elements are essential to everything the neuron does.
In development, the actin filaments form delicate structures called filopodia (literally, “hair-feet”) which sample the environment and pick up cues from the growing neuron’s surroundings, helping to guide it to its final destination. In that way, your developing motor neurons find the muscles they’re supposed to connect to. Taken together, these filopodia form a structure called the growth cone, a name coined by Ramón y Cajal.
The stalk that supports these actin filaments has a core of microtubules for rigidity and strength. The growing ends of microtubules push the entire growth cone forward as the neuron grows, and the microtubules surrounded by a cell membrane will form the axon when the neuron matures.
Axons cut in cross-section show microtubules as little dark circles. These microtubules not only provide structural support, but also play a key role as the “train tracks” on which proteins like kinesin and dynein move as the “engines” moving materials from the cell body to the axon terminal, and from the axon terminal to the cell body. This process is called axonal or axoplasmic transport.
Rod photoreceptors have an elaborate stack of membranes, called the outer segment, which carry the proteins needed to turn light particle-waves (photons) into an electrochemical signal that the neuron can use. This membrane stack, which gives the rod its shape, is connected to the cell body (inner segment) of the photoreceptor by a modified cilium with the cilium’s usual arrangement of microtubules. The microtubules in the rod photoreceptor are shown by the red arrow in this electron micrograph. Note the presence of numerous mitochondria in the inner segment of the rod photoreceptor. This energy is needed to reverse the flow of charged ions through the channels used for visual transduction.