College of Pharmacy
63 Establishing an Extraction Protocol for Amphotericin B from Human Plasma
Evan Nayee and Nitish Khurana
Faculty Mentor: Nitish Khurana (Pharmaceutics & Pharmaceutical Chemistry, University of Utah)
Introduction
Extracorporeal membrane oxygenation (ECMO) is a life support technology that assists or, in extreme cases, temporarily takes over for the heart and/or lungs of critically ill patients. ECMO works by pumping a patient’s blood through an oxygenator that introduces oxygen and removes carbon dioxide before the blood is pumped back into the body. ECMO is used to allow the heart and lungs to heal or to bridge the time before the patient receives a transplant1.
By providing more time for their organs to heal, ECMO saves the lives of patients who otherwise would not survive. In spite of its benefits, using ECMO carries several risks, ranging from excess bleeding or clotting to poor blood flow to the limbs (which occasionally results in amputation). The mortality rate of patients on ECMO is upwards of 40%2. One of the biggest challenges with ECMO is properly dosing medication to patients due to drug adsorption2. Drug absorption is when medication adheres to the tubing or oxygenator fibers due to hydrophobic interactions between these components and hydrophobic drugs, removing a portion of the dose from the patient’s bloodstream. Adsorption makes it difficult to know how much of a drug is in circulation where it can treat its target disease versus how much is adsorbed onto the ECMO circuit and, therefore, not contributing to treatment. Without this information, clinicians struggle to ensure patients get the right amount of medication to manage their condition. Some examples of drugs that are prone to adsorption include propofol, midazolam, lorazepam, and fentanyl3,4.
Amphotericin B (AmpB), a member of the polyene class, is an antifungal medication used to treat invasive fungal infections5. In particular, it is used to treat pulmonary fungal infections, which are a serious morbidity in ECMO patients6,7. Due to its significance in ECMO patients, it is important to determine whether AmpB is subject to adsorption.
Herein, we aimed to determine if adsorption occurs when AmpB is administered to ECMO circuits. In particular, the focus was on developing the extraction protocol to be able to quantify AmpB in the blood samples taken during the studies.
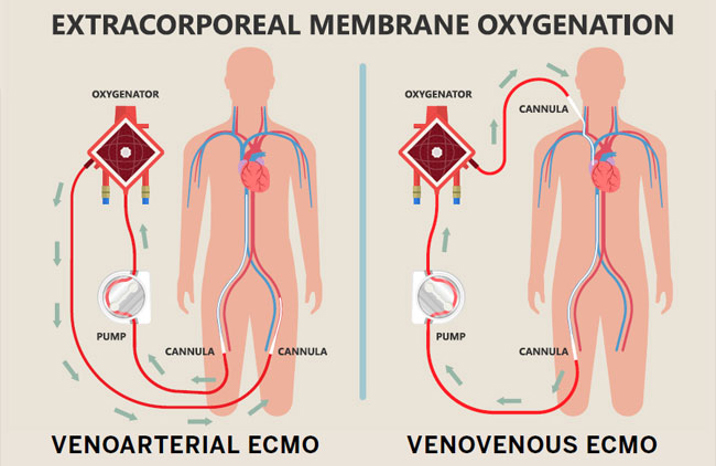
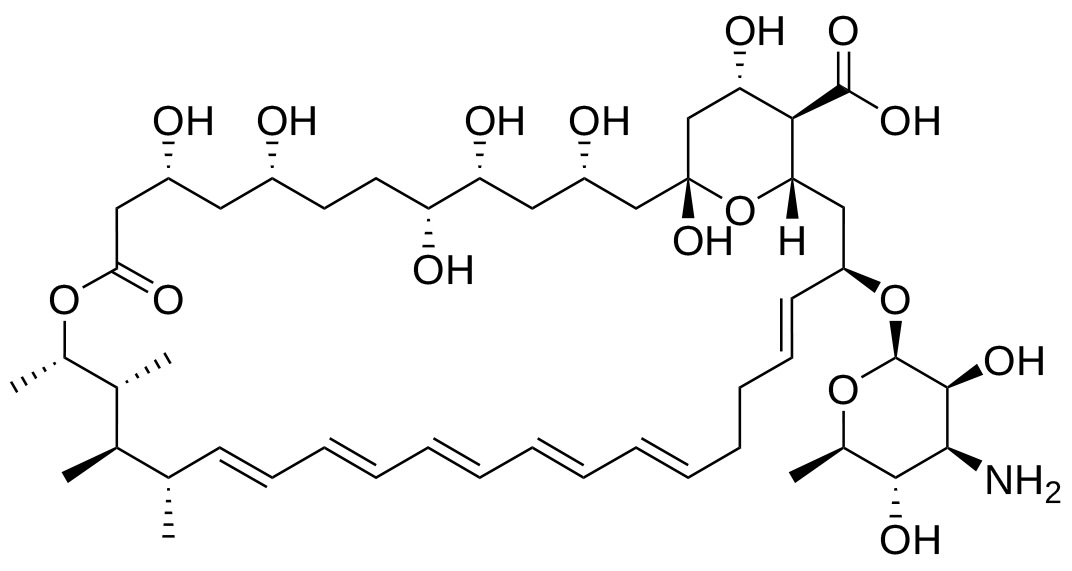
Methods
AmpB was dissolved in Dimethyl Sulfoxide (DMSO) at a concentration of 10mg/mL. A UV absorption spectrum was then run, and it was determined that λmax = 409 nm. AmpB was dissolved in Acetonitrile (ACN) or Methanol (MeOH) at a range of desired concentrations for evaluation with UV spectrometry (Concentration range: 10μg/mL-90μg/mL, intervals of 10μg/mL) or High-Performance Liquid Chromatography (HPLC) (Concentration range: 2μg/mL-10μg/mL, intervals of 2μg/mL).
To evaluate the extraction efficiency of AmpB from human plasma, AmpB in DMSO was then added to human plasma to achieve different concentrations within the same ranges used earlier. 300 μL of plasma was added to an equal volume of ACN or MeOH to crash out the proteins. The solution was then centrifuged at 12,000 rpm for 20 minutes. The supernatant (top liquid) was collected and analyzed using either UV spectrometry or HPLC to quantify the amount of AmpB present in the samples. All experiments were run in triplicate.
UV spectrometry works by passing ultraviolet light through a sample and measuring how much of the light was absorbed by the sample (absorbance)10. Different compounds have unique wavelengths at which they absorb light, so by measuring the absorbance of our samples at the wavelength that AmpB absorbs (409 nm) we are able to get an absorbance value for the sample that corresponds to the concentration of AmpB. The higher the concentration of AmpB, the higher the absorbance value, and vice versa.
HPLC consists of a liquid mobile phase, a stationary phase, and the sample of the compound being analyzed11. HPLC works by mixing the sample with the liquid mobile phase before passing it through the column containing the solid stationary phase. Based on the different properties of the sample (polarity, size, charge), it will have a higher binding affinity for either the mobile phase or the stationary phase. The more the sample binds to the stationary phase, the longer it will take to elute out of the column and be detected, while a sample with a higher affinity for the mobile phase will elute out of the column faster and register with the detector earlier. Based on how long it takes for a sample to reach the detector, it is possible to determine what a compound is, while the area under the curve/peak that is produced when the sample reaches the detector allows the concentration of the compound to be determined using a calibration curve. After much optimization, the mobile phase used was 40% ACN and 60% Potassium Phosphate (KH2PO4) buffer at a pH of 2.5 with a flow rate of 1mL/min. The injection volume was 20 μL with a C18 column heated to 30 °C.
Once the absorbance (UV spectroscopy) or area under the curve (HPLC) was established for the range of known concentrations in both solvent and plasma, the concentration vs. absorbance/area under the curve was graphed to create a calibration curve. The equations from these calibration curves were then used to calculate the concentration in samples of unknown concentration, given their absorbance/area under the curve. The recovery rate of AmpB for the extraction process was also calculated using these calibration curves.
Results
The UV absorption spectrum for AmpB was evaluated, and λmax = 409 nm was determined (Fig. 3). Calibration curves were obtained using UV spectrometry for AmpB in MeOH (R2=0.9892) and ACN (R2=0.9816) (Fig. 4). Calibration curves after AmpB was extracted from human plasma using the solvents were obtained using UV spectrometry: MeOH (R2=0.9729) and ACN (R2=0.8917) (Fig. 5). The lower detection limit (LOD) for AmpB using UV was 10 µg/mL. To further reduce the LOD, High Performance Liquid Chromatography (HPLC) was used to measure concentrations of AmpB in MeOH. A calibration curve was obtained (R2=0.9986) with a LOD of 2 µg/mL (Fig. 6). The recovery rate of AmpB extracted from plasma samples using MeOH was ~90%.
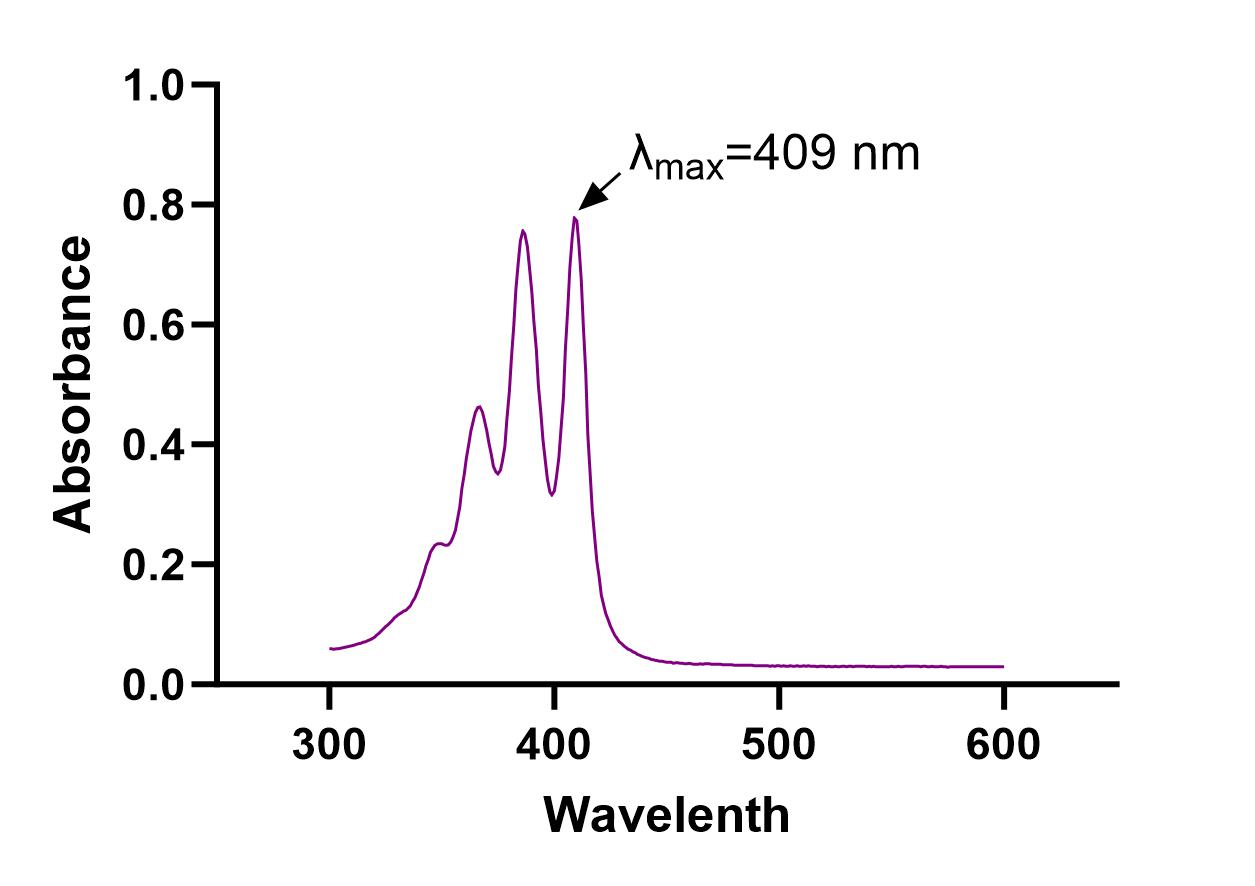
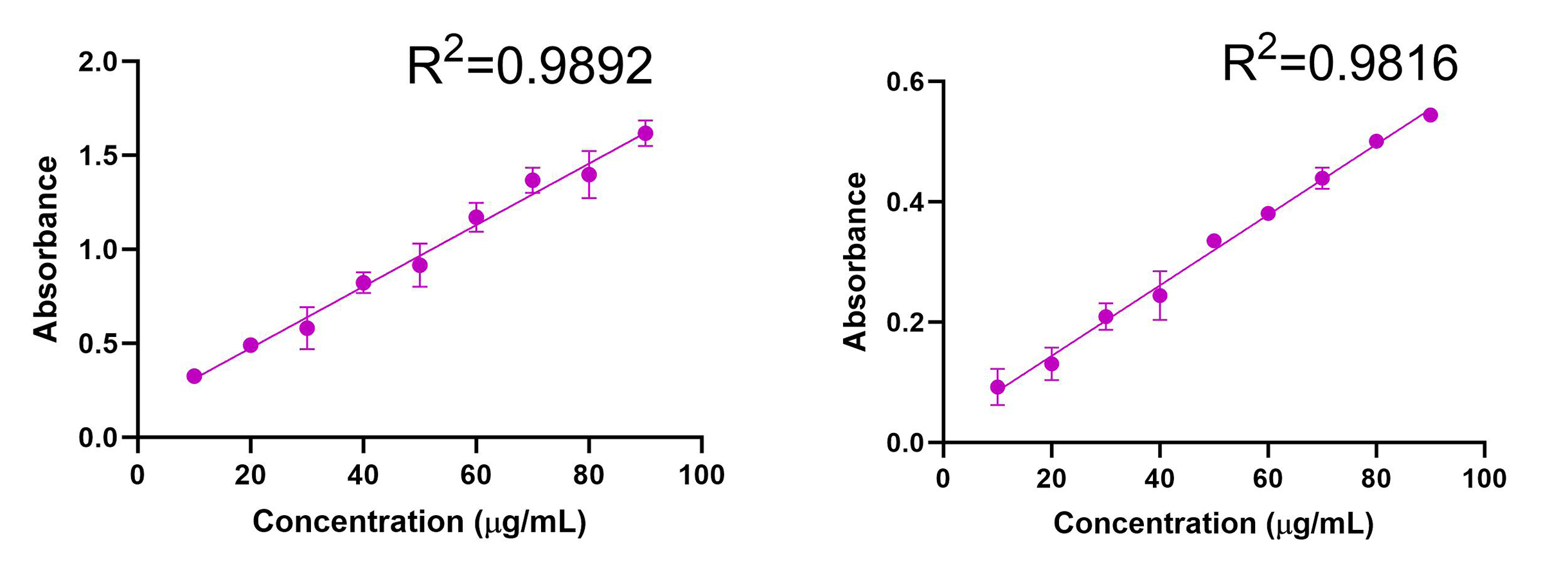
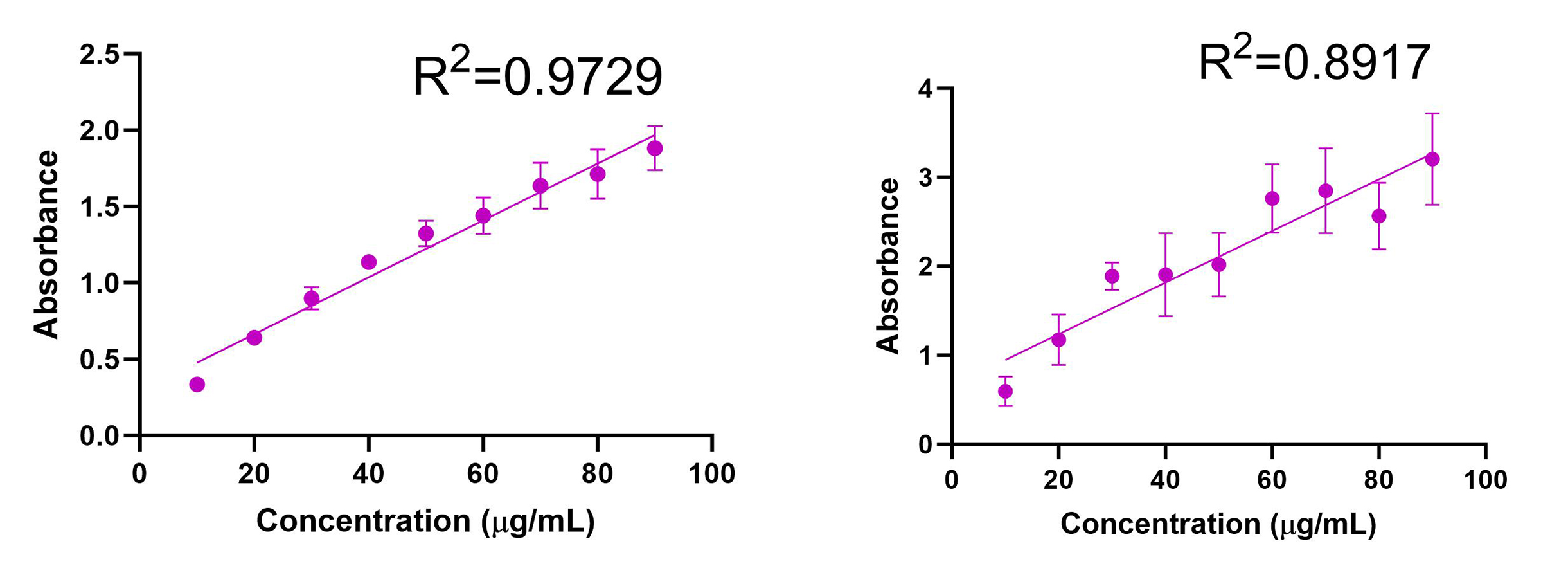
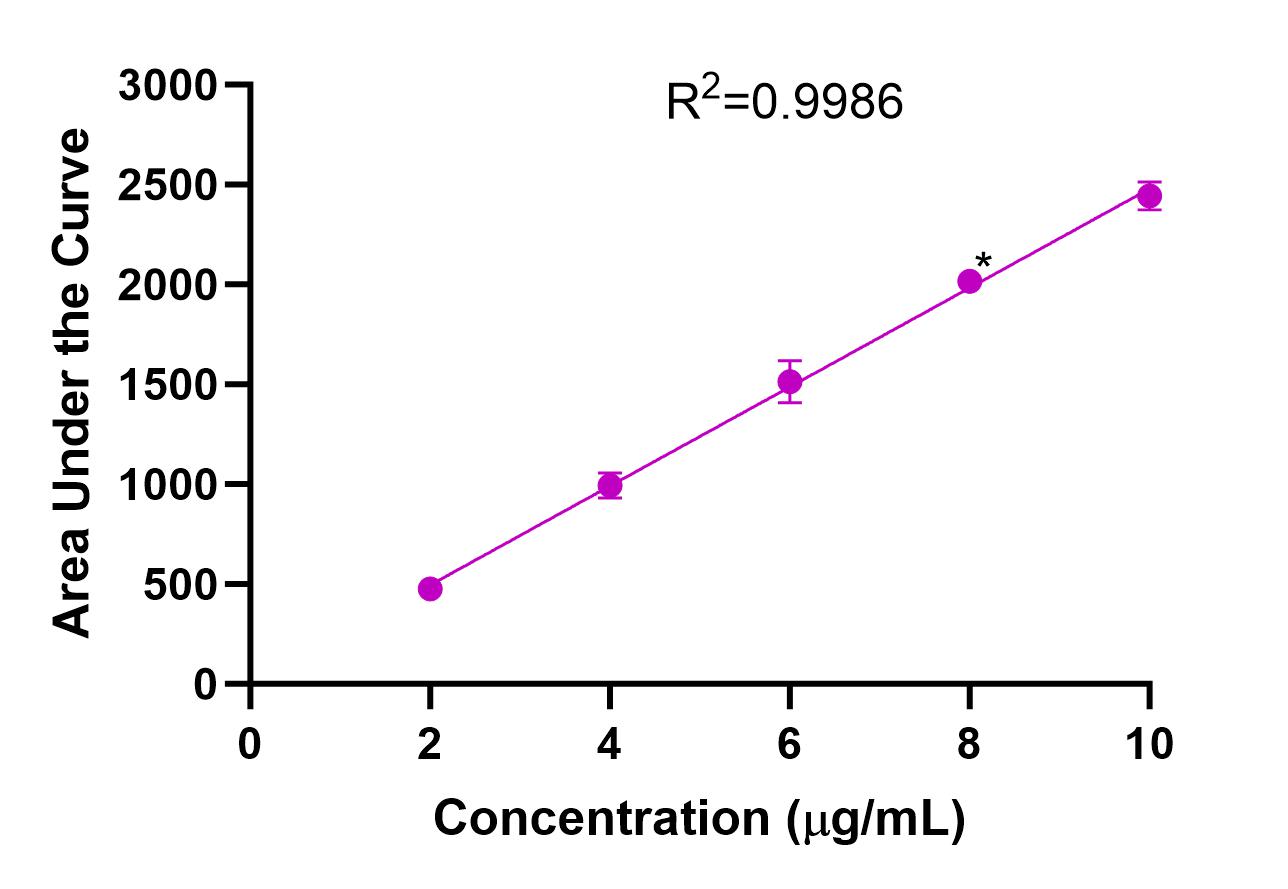
Discussion
Drug adsorption poses a challenge in the treatment of ECMO patients because it hinders clinicians’ ability to know how much medication is actually acting on a patient. An important step to mitigating this issue is understanding which drugs are subject to adsorption, a substantial clinical need that is currently unmet. Knowing the adsorption profile is especially important for medications that are frequently used in ECMO patients, such as Amphotericin B. This project aimed to close that knowledge gap by developing an extraction and quantification method for AmpB that will be used in ex vivo studies to determine its adsorption profile.
We started by determining the wavelength of maximum absorbance for AmpB, which was found to be 409nm. We then established calibration curves for AmpB in both potential solvents that would be used to crash the plasma proteins, selecting 10 μg/mL to 90 μg/mL as the concentration range since those values fell between the minimum absorbance and maximum absorbance values of the UV spectrophotometer. AmpB was dissolved in MeOH (R2=0.9892) and yielded a similar absorbance correlation to ACN (R2=0.9816), meaning that both solvents were viable candidates to test when extracting the drug from plasma since they both produced high-quality lines of best fit. When the solvents were used to extract AmpB from plasma (with the same concentrations 10 μg/mL to 90 μg/mL used for the calibration curves without plasma), MeOH (R2=0.9729) produced a much tighter correlation (and therefore better trendline) than ACN (R2=0.8917). A highly correlated trendline is extremely important since that equation will be used to determine the amount of AmpB present and ultimately measure adsorption in samples of unknown concentrations taken during ex vivo studies. Since MeOH produced more accurate data in the UV spectrometer, we elected to test MeOH only in HPLC.
When AmpB was tested using HPLC, we found that the lower limit of detection (LOD) was 2 μg/mL compared to 10 μg/mL in the UV spectrometer, meaning that HPLC was more sensitive and could detect AmpB at 5-fold lower concentration than the UV spectrometer. Due to this, the concentration ranges tested using HPLC were between 2 μg/mL and 10 μg/mL. AmpB dissolved in MeOH (R2=0.9986) produced a more correlated trendline than the same experiment in the UV spectrometer (R2=0.9892), making HPLC the optimal instrument for evaluating samples during ex vivo studies.
It is important to establish whether any drug was lost from samples during/due to the extraction process since the goal of ex vivo studies is to accurately measure how much drug is present in samples as a means of accurately determining the degree of adsorption. The recovery rate was determined to be ~90%, meaning that minimal drug loss occurred during the extraction process. Since a successful extraction protocol was developed, the next step is to finish validating the protocol by testing plasma samples in HPLC before implementing this protocol in ex vivo studies in order to determine the adsorption profile of AmpB.
Conclusion
There is an unmet clinical need surrounding accurate therapeutic dosage of medications in patients on ECMO cardiopulmonary support. This is largely due to the impact of drug adsorption by ECMO circuits on the amount of medication in the bloodstream and the lack of research on whether medications commonly used in ECMO patients are prone to adsorption. As the first step in studying the adsorption profile of Amphotericin B, which is commonly used to treat fungal infections while on ECMO, we developed a protocol to extract AmpB from human plasma samples using MeOH or ACN before quantifying it using UV spectrometry or HPLC. This protocol was successfully established, with the recovery rate of AmpB being ~90% when extracted using MeOH. The next step is to test the extraction protocol with plasma samples in HPLC to finish validating it before using it during ex vivo studies to determine the adsorption profile of AmpB.
Acknowledgments
This work was supported by SPUR from the Office of Undergraduate Research at the University of Utah, awarded to Evan Nayee. Special thanks to Nitish Khurana, PhD., Abigail Pulsipher, PhD., and Hamid Ghandehari, PhD. for their mentorship and guidance throughout this project.
Footnotes
- Mayo Clinic Staff. (2024, June 15). Extracorporeal membrane oxygenation (ECMO). Mayo Clinic. https://www.mayoclinic.org/tests-procedures/ecmo/about/pac-20484615
- Khurana, N., Watkins, K., Ghatak, D., Staples, J., Hubbard, O., Yellepeddi, V., Watt, K., & Ghandehari, H. (2024). Reducing hydrophobic drug adsorption in an in-vitro extracorporeal membrane oxygenation model. European Journal of Pharmaceutics and Biopharmaceutics, 198. https://doi.org/10.1016/j.ejpb.2024.114261
- Khurana, N., Sünner, T., Hubbard, O., Imburgia, C., Stoddard, G. J., Yellepeddi, V., Ghandehari, H., & Watt, K. M. (2023). Micellar encapsulation of propofol reduces its adsorption on extracorporeal membrane oxygenator (ECMO) circuit. The AAPS Journal, 25(4). https://doi.org/10.1208/s12248-023-00817-2
- Harthan, A. A., Buckley, K. W., Heger, M. L., Fortuna, R. S., & Mays, K. (2014). Medication adsorption into contemporary extracorporeal membrane oxygenator circuits. The Journal of Pediatric Pharmacology and Therapeutics, 19(4), 288–295. https://doi.org/10.5863/1551-6776- 19.4.288
- Drew, R. H. (2023, June 28). Pharmacology of amphotericin B. UpToDate. https://www.uptodate.com/contents/pharmacology-of-amphotericin-b
- de Pablo, E., O’Connell, P., Fernández-García, R., Marchand, S., Chauzy, A., Tewes, F., Dea-Ayuela, M. A., Kumar, D., Bolás, F., Ballesteros, M. P., Torrado, J. J., Healy, A. M., & Serrano, D. R. (2023). Targeting lung macrophages for fungal and parasitic pulmonary infections with innovative amphotericin B dry powder inhalers. International Journal of Pharmaceutics, 635. https://doi.org/10.1016/j.ijpharm.2023.122788
- Jendoubi, A., Pressiat, C., De Roux, Q., Hulin, A., Ghaleh, B., Tissier, R., Kohlhauer, M., & Mongardon, N. (2024). The impact of extracorporeal membrane oxygenation on antifungal pharmacokinetics: A systematic review. International Journal of Antimicrobial Agents, 63(2). https://doi.org/10.1016/j.ijantimicag.2023.107078
- Mathew, J. C. (2021). Extra Corporeal Membrane Oxygenation. For Physicians – ECMO: Life Support Option. SingHealth DukeNUS. Retrieved July 15, 2024, from https://www.singhealthdukenus.com.sg/news/patient-care/for-physicians-ecmo-life-support-option.
- Tan, T. R., Hoi, K. M., Zhang, P., & Ng, S. K. (2016). Characterization of a polyethylene glycol- amphotericin B conjugate loaded with free AMB for improved antifungal efficacy. PLOS ONE, 11(3). https://doi.org/10.1371/journal.pone.0152112
- Raja, P. M. V., & Barron, A. R. (n.d.). 4.4: UV-visible spectroscopy. Chemistry LibreTexts. https://chem.libretexts.org/Bookshelves/Analytical_Chemistry/Physical_Methods_in_Chemistr y_and_Nano_Science_(Barron)/04%3A_Chemical_Speciation/4.04%3A_UV- Visible_Spectroscopy
- Thermo Fisher Scientific. (n.d.). HPLC Basics. Thermo Fisher Scientific – US. https://www.thermofisher.com/us/en/home/industrial/chromatography/chromatography-learning-center/liquid-chromatography-information/hplc-basics.html
Media Attributions
- 137489262_figure_1
- 146879582_figure_2
- 146879585_figure_3
- 146881378_figure_4
- 146881870_figure_5
- 146882801_figure_6