Anterograde and Retrograde Transport Mechanisms in Neurons
Alivia Deimer
This is a first draft which has not been edited. If you have questions, or want to help in the writing or editing process, please contact the author: aliviadeimer@mail.weber.edu
Introduction to Axonal Transport
Just like a car needs various fluids to run– like oil, gasoline, and coolant– our nervous cells require a variety of materials to maintain function. While many materials can diffuse into cells along concentration gradients as needed, other packs of materials are far too large to move throughout cells in this way.
These packages are often actively transported by proteins kinesin and dynein along microtubules.
This transport can occur in the anterograde (plus-ended) direction, which transports cargo from the cell body to the periphery of the cell, or in the retrograde (minus-ended) direction, which moves from the periphery of the cell towards the cell body.
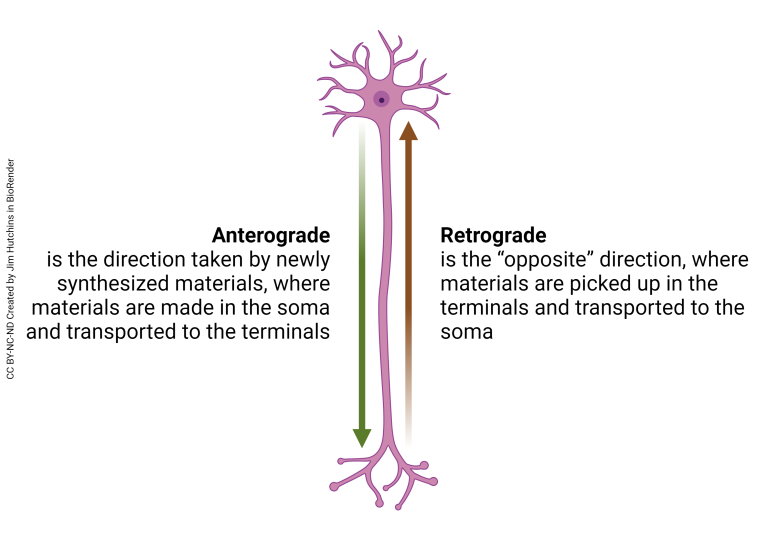
Kinesin
Kinesins are a family of proteins that use adenosine triphosphate (ATP) to “walk” along microtubules and actin filaments to transport intermediate filaments, ribonucleoprotein particles, and vesicles away from the soma (cell body) of the neuron.
Both dynein and kinesin work as the workhorses of the cell that drive axonal transport in the anterograde and retrograde direction. In the case in kinesin, it has two “heads” that walk along the microtubule (shown in the video below), one that binds with the microtubule, and one that binds with ATP. When one head binds with ATP, it uses the energy from
the ATP hydrolysis to flip over the other, binding to the microtubule farther down the assembled “road”. This binding action results in the “walking” motion observed in kinesin proteins.
Kinesin molecules have a stalk and a tail– when binding to cargo, specific proteins inside of the tail attach to the package, holding strong to keep hold of the cargo while the heads walk along the microtubule. Kinesin can carry cargo many times its size, similar to how ants can carry ten times their weight when transporting food to the colony.

Dynein
Dynein proteins work in tandem with kinesin proteins to transport cargo along microtubules. In axonal transport, cytoplasmic dynein is responsible for intracellular transport of packages across microtubules– this process is quite similar to kinesin’s “walking” motion, but has a few key differences.
Dynein, like kinesin, has a heavy chain at the bottom which interacts with the microtubule. Unlike kinesin, though, it does not enact this process alone– another protein complex called dynactin works to activate dynein and aid it in moving packages across long intracellular distances. In fact, dynein has larger steps than kinesin, allowing it to move packages along the microtubule quicker than kinesin. Also unlike kinesin, dynein can move in both the anterograde and retrograde directions.
Dynein has six AAA+ domains, putting it into the class of AAA motor proteins– ATP binding and hydrolysis (which drive movement in dynein similarly as to kinesin) mainly occur at the AAA1 area of the complex.
This hydrolysis of ATP results in dynein walking on a microtubule similarly to kinesin.
Anterograde Transport
As stated before, anterograde transport has motor protein cargo being transported away from the cell body. Most of our anterograde transport is conducted by kinesin proteins, which walk along microtubules towards our axon terminals. This type of transport is often called plus-ended transport because microtubules are organized to have their positive ends towards the axon terminal, and their negative ends towards the cell body. As such, as kinesin walks towards the axon terminal, it is moving towards the positive end of the microtubule.
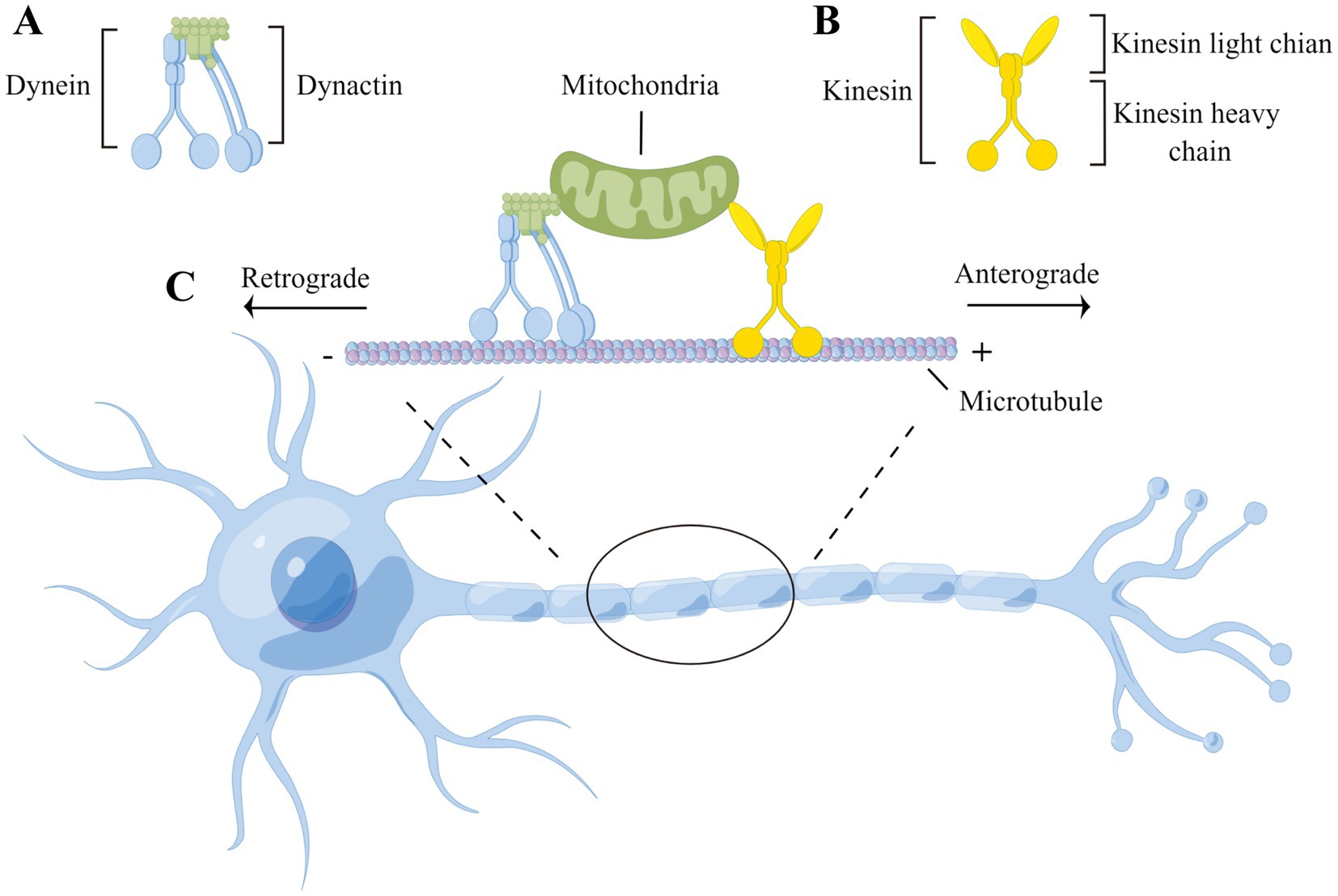
Material moved in the anterograde direction tends to be small, but numerous. Anterograde transport can vary between fast and slow speeds– while neurotransmitters, membrane proteins, lipids, and mitochondria fall within the fast transport category, microfilaments and neurofilaments tend to be transported slower along the microtubule.
Retrograde Transport
Retrograde transport sees packages being transported toward the cell body, traveling towards the negative side of the microtubule. Retrograde transport is largely conducted by dynein. The packages carried by dynein tend to be larger, carrying proteins and exogenous material back towards the cell
body. Because of dynein’s larger “steps”, retrograde transport tends to have a faster transport rate on average than anterograde transport.
Retrograde transport can also act as a sort of “report” to the cell body about the condition of the axon terminals, which reveals its smaller role in the communication network of the cell.
How is Transport Halted?
When kinesin or dynein arrives at its location, it must detach the goods that it was carrying to complete its mission. This begs the question, though, of how these motor proteins know where to stop moving.
The answer lies in the make-up of the microtubule on which kinesin and dynein travel along. Along with having charged ends, microtubules also have specific binding sites. These binding cites allow for these motor proteins to connect to the microtubule and walk along it, and specific cites also function as a sort of stop sign for kinesin and dynein, signaling them to separate from their cargo.
When a motor protein reaches the end of a microtubule, it will encounter one of these “stop sign” binding sites– binding to this site sparks a conformational change in the motor protein, which is
known as that motor protein’s detachment mechanism. The change in shape triggered by the binding site weakens the protein’s hold on its cargo, which results in the cargo detaching from the motor protein and joining whichever cellular location was the end-point for transport.