Sensory Systems | Vestibular (Balance) System
Objective 6
For the vestibular system, define static equilibrium. Define dynamic equilibrium. Explain the encoding of static equilibrium in the maculae. Explain the encoding of dynamic equilibrium in the semicircular ducts and the ampullae. Describe the pathways taken for vestibular sensation information from the semicircular canals to brain.
Balance depends on knowing where our bodies lie in relationship to gravity. The vestibular system is responsible for calculating our position and motion in relationship to the direction that gravity pulls us. In the absence of gravity, that calculation becomes disrupted and space sickness results. If the visual system and vestibular system disagree on the direction of the horizon, then motion sickness or seasickness result.
Like a plane, the head can move in three dimensions. For a plane, these three dimensions are termed “roll”, “pitch”, and “yaw”.
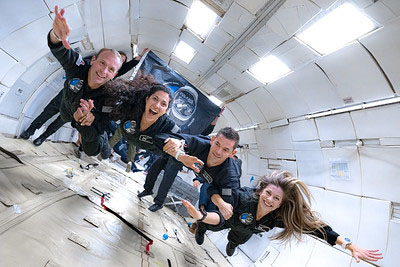
There are two important features of our relationship to gravity that need to be calculated:
- static equilibrium, or head tilt, which is called linear acceleration by engineers. This is not movement but rather a readout of the head position compared to the direction of gravity.
- dynamic equilibrium, or head rotation or head movement, which is called angular acceleration by engineers.
Static Equilibrium
Static equilibrium, or head tilt, is coded in receptive organs called maculae (singular macula, Latin, “spot”). Maculae are found in two enlargements of the semicircular canals, the utricle and saccule (Latin: “leather bag” and “little sack”).
The maculae contain hair cells which closely resemble the hair cells of the cochlea (there are minor differences that we won’t discuss here).
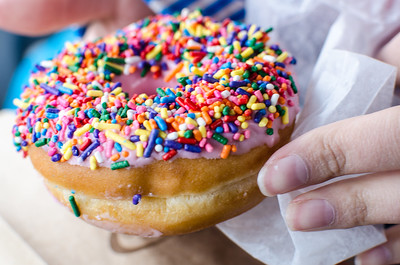
The stereocilia of macular hair cells, however, are embedded in a gelatin-like otolith membrane with otoliths on top like sprinkles on a frosted donut.
The otoliths (Greek οτο-, oto–, “ear”+ -λιθος, –lithos, “stone”) are just what the name suggests: crystals of calcium carbonate which slide on top of the hairs and move them to and fro. As the head tilts, the otoliths start a landslide in the gelatinous goo that surrounds the stereocilia, and this bends the cilia, which pulls on tethers, which causes ion channels to open and sets up the potential changes that alter neurotransmitter release onto the primary afferent fiber.
As in the cochlea, the hair cells do not send out axons. Rather, they make synaptic contact onto the dendritic processes of bipolar cells. The cell bodies of these primary afferents are in Scarpa’s ganglion and their axons are in the vestibular division of CN VIII.
Dynamic Equilibrium
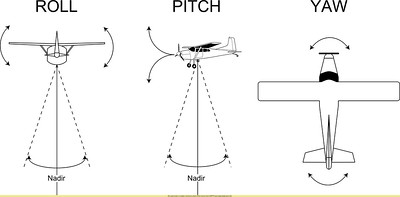
Movement of the head in space (angular acceleration) is the relevant stimulus for the semicircular canals. There are three semicircular canals and three planes of movement (X, Y and Z-axes, or what pilots call roll, pitch and yaw). Each canal is in an optimal position to detect movement in one of the three cardinal planes.
Each ends in an ampulla, where mechanoreceptive hair cells are found.
Imagine a glass full of iced lemonade. As you twirl the glass, the ice and lemonade stay in place while the glass moves. In the same way, the fluid inside the semicircular canals stays in the same place — a property called inertia — while the walls of the semicircular canals move along with the head.
As the fluid rushes past the receptive cells in the ampulla, it bends the hairs and sends a signal that the head is rotating.
The vestibular system uses four vestibular nuclei in the brainstem, but it’s not important for us to learn much about them. It is important to know that the vestibular information is shared with the other sensory systems, particularly with the visual system (to coordinate head and eye movements) and with proprioceptive systems of the head and neck, to tell the muscle sensors what direction gravity is coming from. We will look at this vestibulo-ocular reflex in Objective 10.
A small part of the insula, called the parieto-insular vestibular cortex (PIVC), is thought to represent the location of vestibular cortex.
Media Attributions
- U12-048 EarAnatomy_InternalEar © BruceBlaus is licensed under a CC BY (Attribution) license
- U12-061a Roll Pitch Yaw © Beck, Ant is licensed under a CC BY (Attribution) license
- U12-059a Zero-gravity Research Flight © Polaris Program / Kraus, John is licensed under a CC BY-NC-ND (Attribution NonCommercial NoDerivatives) license
- U12-060 Vestibular Macula © Betts, J. Gordon; Young, Kelly A.; Wise, James A.; Johnson, Eddie; Poe, Brandon; Kruse, Dean H. Korol, Oksana; Johnson, Jody E.; Womble, Mark & DeSaix, Peter is licensed under a CC BY (Attribution) license
- U12-060a Sprinkle Donut © m01229 is licensed under a CC BY (Attribution) license
- U12-061 Vestibular Semicircular Canals © Betts, J. Gordon; Young, Kelly A.; Wise, James A.; Johnson, Eddie; Poe, Brandon; Kruse, Dean H. Korol, Oksana; Johnson, Jody E.; Womble, Mark & DeSaix, Peter is licensed under a CC BY (Attribution) license
- U12-061a Pitch Roll Yaw © Beck, Ant is licensed under a CC BY (Attribution) license
- U12-062 Sensory Homunculus © Betts, J. Gordon; Young, Kelly A.; Wise, James A.; Johnson, Eddie; Poe, Brandon; Kruse, Dean H. Korol, Oksana; Johnson, Jody E.; Womble, Mark & DeSaix, Peter adapted by Jim Hutchins is licensed under a CC BY (Attribution) license
- U12-063 PIVC © Carter, Henry Vandyke adapted by Jim Hutchins and Alexa Crookston is licensed under a CC BY-SA (Attribution ShareAlike) license