Myelination and Regeneration of Neurons
Objective 7
Discuss how the myelination of axons changes their electrical properties.
The nerve cell axon, and many dendrites, are long tubes that are negatively charged inside. If there are no or few ion channels, it acts like a wire, with passive spread of current in the form of positively-charged K+ ions spreading away from the site of depolarization. The positively-charged sodium ions enter through the voltage-gated Na+ channels and repel K+ ions in the region of the voltage-gated channel. As the K+ ions are repelled away from the entering Na+, they form a current.
Because of the presence of leakage channels, this current spread eventually decrements to a point of near-nothingness. This was illustrated in the previous chapter.
In some axons and dendrites, this is a feature of how they work. For example, in dendrites, the decrement in electrical energy is used to transform information flow in a process called temporal and spatial summation.
If the neuron needs to send information over a long distance, however, then it becomes a problem. Either the axon has to be completely coated with voltage-gated sodium and potassium channels, or we can space them out but stop current loss in between the patches that have no channels. In that case, as seen in the purple line in the graph at right, a sheath of lipid called myelin acts as an insulator to decrease the amount of current loss. Axons that carry a myelin sheath are called myelinated.
In the central nervous system (CNS), myelination is the responsibility of oligodendrocytes. In the peripheral nervous system (PNS), myelination is the responsibility of Schwann cells. At the end of this objective, we’ll see how these different glial cell types are reflected in a difference in the capacity for axon repair after nerve damage.
Axons that are myelinated conduct action potentials faster than unmyelinated axons.
Axons that are larger conduct action potentials faster than smaller axons.
This diagram is longitudinal (lengthwise) cross-section through an axon. To the right and the left are myelin sheaths. In the center is a gap in the myelin sheath called the node of Ranvier.
We saw that the action potential depends on the coordinated action of voltage-gated Na+ channels and voltage-gated K+ channels. That supposes that ions (Na+ and K+) can move through those channels. But ions can’t penetrate the myelin sheath, so no action potentials can be generated in those regions of the myelinated axon. The only movement of ions occurs within the “wire” of the axon through passive spread, as discussed in the previous objective.
So we put all of our voltage-gated Na+ channels and voltage-gated K+ channels at the node of Ranvier. Now between the nodes, where myelin covers the axon, there is rapid passive spread of current. The axon is “tuned” to its length constant so that by the time the passive current spread reaches the next node, there’s just enough potential energy (voltage) left to trigger a new action potential (i.e. it just barely reaches threshold at that point). That causes the positive feedback loop or chain reaction in the voltage-gated Na+ channels and a new action potential is fired at the node. That spreads to the next node passively, and so forth.
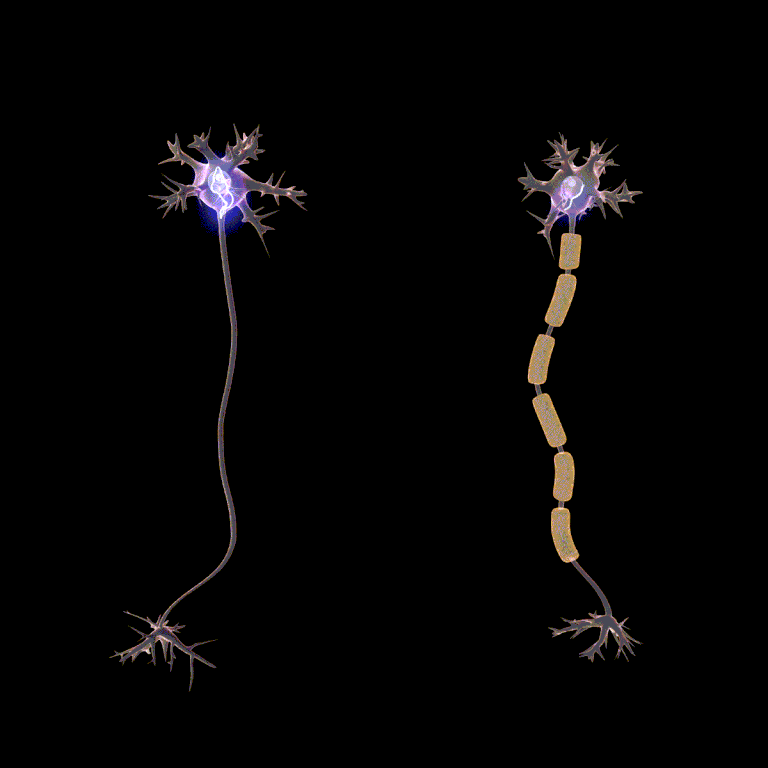
Passive current spread is very fast, at maybe one-millionth the speed of light. But that’s still 3000 m/sec. Now we get to the node, and we have to wait … wait … about a millisecond or two for the voltage-gated Na+ channels and the voltage-gated K+ channels to do their thing. Then we rapidly spread to the next node, and in this way we transmit the action potential from one end of the axon to the other.
Because looking at this from outside the axon, the action potential appears to “jump” from one node to the next, this mode of action potential spread is called saltatory conduction (Latin, saltere, “to hop; to dance”).
The preceding is all you need to know for this objective. But to help you relate these concepts to human medicine, let’s (optionally) consider two pathological situations that affect the myelin sheath.
In some patients, for reasons that are not at all understood, the body’s defenses decide to mount an autoimmune attack on the myelin sheath. As the myelin sheath is attacked, the attacking cells attract water and clinicians can see the areas of attack by visualizing this increased water using magnetic resonance imaging.
Recall that the nodes are spaced precisely so that the passive current spread to the next node is just large enough to trigger a new action potential. But if the myelin sheath is stripped away or damaged, ions leak out through the uninsulated axon and when the passive current spread reaches the next node, it’s too small to trigger a new action potential. In this way, the axon is unable to reliably pass action potentials from the initial segment to the axon terminals.
One major difference between CNS axons and PNS axons is that CNS axons cannot regenerate, while PNS axons can. The functional difference between these two areas is the difference in the glycoproteins residing on the surface of oligodendrocytes and Schwann cells. Oligodendrocytes of the CNS do not support axon growth. Schwann cells of the PNS do. It’s more difficult to heal from a nerve crush than from a clean slice through the nerve. In a clean slice through a PNS nerve, the fibroblasts (spindle-shaped brown cells) and the immune cells (round purple cells) secrete chemical factors that signal the remaining Schwann cells to support regrowth. This change in Schwann cell status can be seen through the microscope and the areas of activated Schwann cells are called Büngner bands.
If you have never cut yourself so badly that the area supplied by a nerve branch becomes numb, consider yourself lucky. But if you know someone who has, ask them what happens next. Nerves regrow at about 1 mm/day. It may take days, or weeks, or months, but damage to peripheral nerves (such as in the extremities) usually results in a slow restoration of nerve axon function as they regrow from the cut stump. In the CNS, regeneration never happens and the function is lost forever.
Media Attributions
- U13-044 Axon At Rest © Crookston, Alexa is licensed under a CC BY-SA (Attribution ShareAlike) license
- U13-039 Membrane Potential and Depolarization © Barnett, Cierra Memphis is licensed under a CC BY-NC-ND (Attribution NonCommercial NoDerivatives) license
- U13-045 Myelin Sheath Formation © Crookston, Alexa is licensed under a CC BY-SA (Attribution ShareAlike) license
- U13-046 Myelinated Axons © Deerinck, Tom and Ellisman, Mark, National Center for Microscopy and Imaging Research (NCMIR) is licensed under a CC BY-NC (Attribution NonCommercial) license
- U13-047 Saltatory Conduction © Cornelius, Emma is licensed under a CC BY-NC-ND (Attribution NonCommercial NoDerivatives) license
- U13-048 Saltatory Conduction © Dr. Jana is licensed under a CC BY (Attribution) license
- U13-049 Multiple Sclerosis © Cornelius, Emma is licensed under a CC BY-NC-ND (Attribution NonCommercial NoDerivatives) license
- U13-050 Schwann Cells © Cornelius, Emma is licensed under a CC BY-NC-ND (Attribution NonCommercial NoDerivatives) license