Cells and Molecules of Immunity
Objective 9
Describe the cells and molecules which make up the main components of the immune response.
Cells of the Immune System
This image shows the cells of the immune system. Some of these you met already (e.g. the fibroblast in Unit 7) or will soon (the endothelial cell lining blood vessels in Unit 16).
In Objective 4, you met the granulocytes. They’re in the left-hand column.
- basophil
- eosinophil
- neutrophil
You also met the agranulocytes:
- macrophage
- lymphocytes
- B cell
- plasma cell, the active, antibody-secreting form of the B cell
- natural killer cell
- T cell
- B cell
We will also discuss two cells that have specialized roles in the immune system:
- dendritic cell
- mast cell
Also illustrated here are two cells that will play supporting roles but are not technically part of the immune system:
- endothelial cells, a squamous cell that lines the inner surface of all blood vessels
- fibroblasts, who we met in Unit 7 as the main cell type of connective tissue
Antigen-Presenting Cells
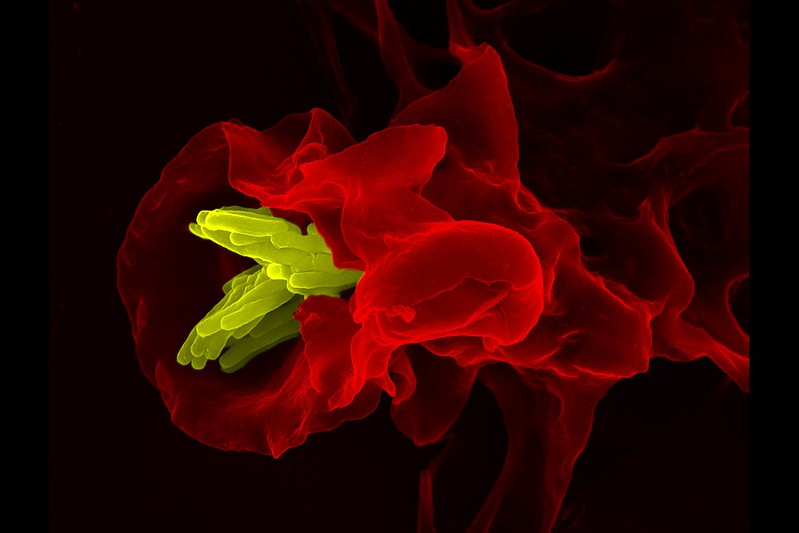
As we will see later, the immune system would rather deal with bite-size pieces of steak rather than trying to digest a whole side of beef. Chopping up the invaders into bite-size pieces is the job of antigen-presenting cells (APCs).
Like a parent making an airplane out of an unpleasant food on a fork, the antigen-presenting cells display pieces of the invaders in a way that makes them attractive to the T cells. This is called, of course, antigen presentation.
Macrophages are in a great position to act as antigen-presenting cells. As the garbage collectors of the body, they’re already patrolling the connective tissues and looking for things to eat and chop into pieces. If one of these pieces is “interesting” to the immune system, it will be presented to a T cell for its review and approval.
Dendritic cells, also derived from monocytes, act as APCs also. They visit sites of inflammation (“war zones”) and take “surveillance photos” of enemies. Then, they present these surveillance photos (antigen fragments) to the other cells of the immune system, particularly T cells, in a nearby lymph node.
B lymphocytes are called “naïve” when they have not yet encountered the antigen which they’re specific for. Once B lymphocytes are experienced, they can also act as APCs.
APCs use a specialized form of an eating process we first saw in Unit 4, phagocytosis (literally, “eating cell process”).
Phagocytosis involves a number of steps that are mostly logical if you remember phagocytosis means cell eating. Just think of the last time you walked into a kitchen when something tasty was being prepared. The smell led to you to the food, you reached out and brought the food to your mouth, chewed it up and swallowed it. Hopefully you didn’t show off your chewed food to family members or friends, but for the sake of the analogy, let’s pretend you did. As you shout “see food!” and open your mouth, you’re presenting what you ate. That’s antigen presentation.
Lymphocytes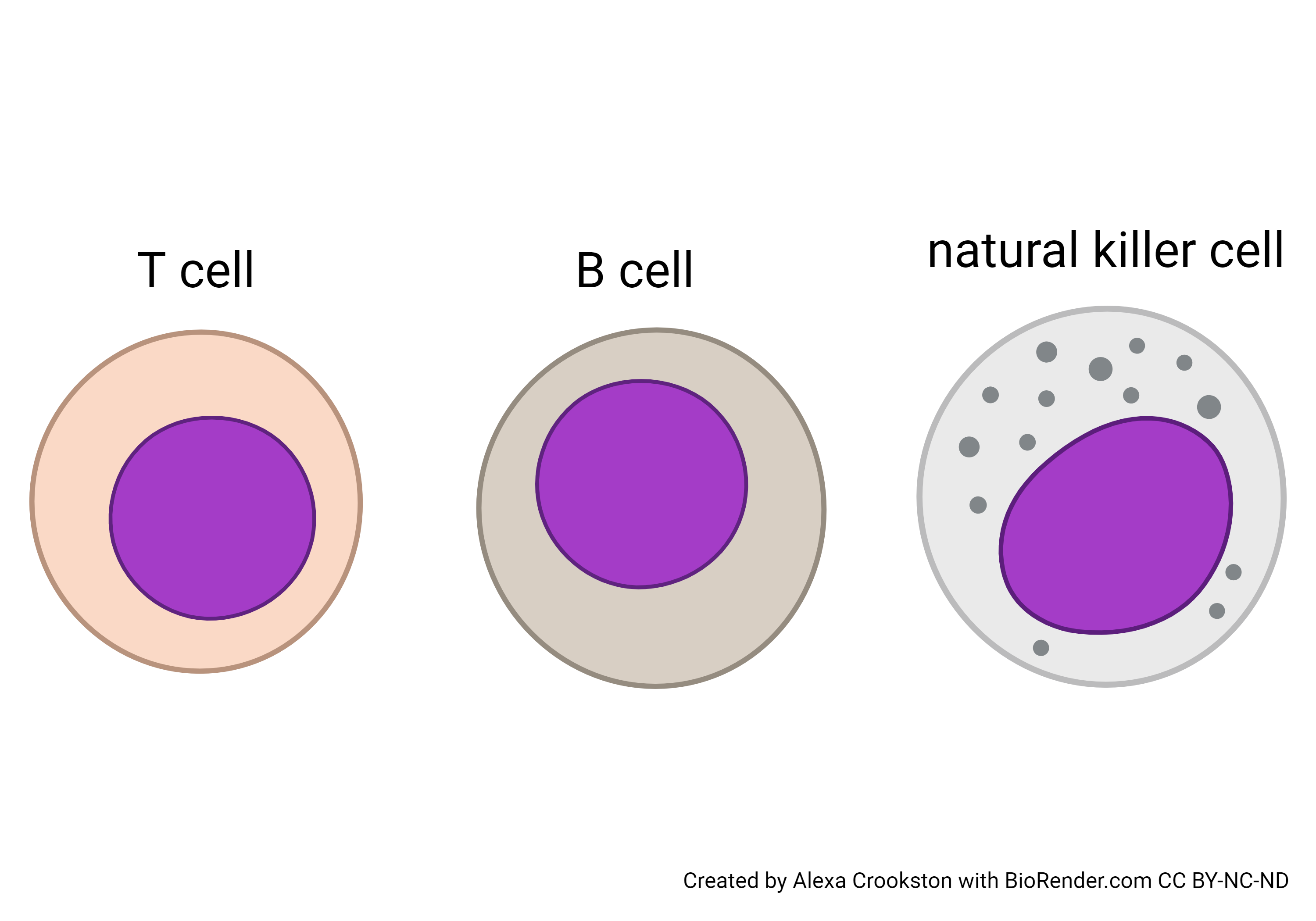
Lymphocytes come in three varieties:
- Lymphocytes which are born in the bone marrow and mature in the thymus, and are therefore called T cells.
- Lymphocytes which are born in the bone marrow and mature in the bone marrow, and are therefore called B cells.
- Lymphocytes which are born in the bone marrow and train with a sensei to become assassins, and are therefore called natural killer (NK) cells. (I’m joking about the sensei, of course, but not about the assassination part.)
We will consider each of these lymphocytes in turn.
T Cells
T cells coordinate different aspects of the immune attack, as shown in the images and in the table below. While other cells work semi-independently, T cells are notable for their ability to cooperate and coordinate their attacks using chemical signals called cytokines. They are also good at recruiting the other cell types of the immune system using cytokine signaling. We will discuss cytokines in detail later in this objective.
T helper (TH) cells are the event planners of the immune system. They talk to everyone, and bring everyone together. The other cells of the immune system do exactly what the T helper tells them to do.
T cytotoxic (TC) cells are also called T killer cells, and killing is what they do. They’re not as elegant as the natural killer cells, but they get the job done with a little bit more brutality and a lot less finesse.
T regulator (TR) cells are poorly understood but they must be very important. They are the peacemakers of the immune system, and without them, we would attack our own body tissues and destroy ourselves with autoimmune diseases. They used to be called T suppressor cells because they suppress the immune response.
The table below lists the important features of each type of T lymphocyte.
B Cells
B cells are the antibody-secreting cells of the body. Like a downtown restaurant, they display their wares in a public way so everyone knows what they’re making. These displayed antibody molecules are called “B cell receptors” (BCRs) in this context. They work just like antibodies, with two antigen binding sites; they’re shaped just like antibodies; and they have a heavy and a light chain, just like antibodies.
When the BCR encounters the antigen it’s made for, two transmembrane proteins called CD79α and CD79β, which sit like sidecars next to the constant (Fc) region of the BCR, detect the binding of antigen to the BCR, convert tyrosine residues in the CD79 protein to phosphotyrosine, and transmit that signal to the interior of the B cell. Through a complex set of chemical signals inside the cell, the B cell is then stimulated to divide.
Active, dividing B cells have one of two fates.
- They can become plasma cells, that activate their protein synthesis machinery (rough endoplasmic reticulum) and pump out (in technical terms) a metric crapton of antibody molecules into the fluid that surrounds them. Generally, this is blood or lymphatic fluid, but can also be mucus, saliva, tears, sweat, or any other body secretion. Plasma cells receive cytokine signals from a T helper cell that tell them what class of antibody to produce (IgG, IgM, IgA, or IgE).
- They can become B memory cells. There are about 100,000,000 different “pocket” shapes representing 100,000,000 different antibody specificities. There are about 3,000,000,000 B cells. In other words, an average of about 30 B cells with a particular pocket shape have to surveil the entire human body, looking for invaders that fit their pockets. It can take a long time to locate the right B cell, on average, about a week, as we will see in Objective 12. We can speed this process up by increasing the number of B cells after the first exposure to an antigen. After all, if you’ve seen the common cold virus once in your life, you’re likely to see it again day after day.
Natural Killer Cells
Natural killer cells are 5-10% of the circulating lymphocytes. Each cell of the body has a “self-destruct” switch called the apoptotic pathway. When this genetic switch is activated, the cell breaks its DNA into small parts and fuses its organelles and cytoplasmic proteins with lysosomes. This recycles as much of the cell as possible. The indigestible part left behind is consumed by a macrophage.
If a cell is cancerous or infected with a virus, it’s likely that its DNA has been altered. That’s why it’s handy to have natural killer cells around. They ensure the infected or transformed DNA does not get loose and harm other cells nearby.
Molecules of the Immune System
CD Markers
Similar to ABO and Rh antigens on the surface of red blood cells, white blood cells also have numerous surface molecules. These surface molecules, most of which are glycoproteins, perform critical functions such as recognition and communication with other cells. The surface molecules can also assist in identifying different subtypes of white blood cells in the body. Because they are used to categorize cells into different groups, they have the strange, but appropriate name “cluster of differentiation” or CD for short.
CD markers on T cells are especially useful in distinguishing the different subtypes. For example, almost all T cells have the CD3 protein, which they use for antigen recognition, but T helper cells also have the CD4 marker. A subgroup of T cells called T cytotoxic cells have CD3, but also have a CD8 marker. These CD markers are used clinically to identify and count cells. The Human Immunodeficiency Virus (HIV) causes destruction of T helper cells (TH cells are CD4+), which can lead to the disease Acquired ImmunoDeficiency Syndrome (AIDS). An important step in making the diagnosis of AIDS is to count the CD4+ T cells a person has.
MHC Markers
You’ll recall that proteins produced by cells are done so by using pieces of DNA called genes. The major histocompatibility complex (MHC) is a group of genes that codes for a group of proteins on the surface of cells. These proteins are sometimes called MHC molecules or human leukocyte antigens (HLA). Originally, they were thought to exist only on leukocytes, so the name HLA fit. Now it is known that they are present on all nucleated cells. These molecules are very important in cell recognition as well as the processing and presenting of antigens. They allow a cell to respond to an antigen directly or to present the antigen to other components of the immune system.
There are two major types of MHC molecules used by the immune system, class I and class II. MHC class I molecules play an important role in defense against viruses. Because viruses target cells with nuclei, it is logical that all nucleated cells would have the ability to make MHC class I proteins. These proteins act like banners that broadcast the message “hey, I’m infected, come take me out!”
MHC class II proteins are used to present antigens from something a cell has engulfed, so they are present on phagocytic cells. MHC class II banners say “Hey, I just ate this, come help me!”
Growth Factors & Cytokines
Recall that we saw this diagram of hematopoiesis in Objective 2, and noted that chemical signals controlled the process of differentiation in blood cells. For example, the development of red blood cells is under the influence of a chemical signal called erythropoietin. Similarly, the cells of the immune system are prompted to reproduce, move, grow, and eat by chemical signals.
The interaction of all players in the immune response is very complicated. Information needs to be passed between cells and organs in order to coordinate the most effective response. This is done through the release of chemical messages called growth factors and cytokines.
Growth factors and similar chemical signals used by lymphocytes are usually called by the special name cytokines. Their functions include cell proliferation in the bone marrow, activation of circulating immune cells, activation of tissue specific macrophages, increased viral resistance and regulation of responses. Each type of cytokine has an immune cell origin and specific function.
Cytokines are further divided into several classes. The major classes of cytokines are:
- interleukins (“IL-“ followed by a number, e.g. IL-1, IL-2, etc.)
- interferons (IFN-α, IFN-β, and IFN-γ)
- colony-stimulating factors (CSFs)
Antibodies
Antibodies are commonly called immunoglobulins (Ig). They have a unique structure that provides for their specificity. The basic antibody structure consists of 4 polypeptide chains, two long (heavy chains) and two short (light chains). Disulfide bonds between amino acid cysteine residues link the chains together. This simple arrangement gives the antibody a characteristic Y-shape. Most antibodies are one Y-shaped unit (monomer), but some can contain multiple units (dimer and pentamer).
There are two main regions of an antibody, the constant region and the variable region. The constant region differs slightly for the different classes of antibodies, but is constant enough to be recognized by other immune system components like macrophages and complement. All humans have essentially the amino acid sequence in the constant region, which is why it is called “constant”, after all. We can engineer an antibody made in mice by clipping off the mouse constant region and attaching a human constant region and use this as a therapeutic drug (these drug names end in “-mab” which is short for “monoclonal antibody”).
The variable region consists of the distal segments of the heavy and light chains and forms the antigen-binding site. Alterations in the protein structure of this portion give the antibody its antigen specificity.
A gene region which can be swapped into or out of a gene is referred to as a gene cassette. Through a process of gene cassette shuffling, called V(D)J recombination, there are 100,000,000 unique pockets created at the tips of each Y-shaped molecule. In other words, your body can recognize 100,000,000 different antigens, and we create these antigen pockets “blind” through randomly shuffling these three types of gene cassette (V, D, and J) and then editing them into a single exon.
In the diagram (which is in a blue box, and not to be learned, just admired), note that the gene shuffling in both the heavy and light chain variable regions (VH and VL) and that all these different genes contribute a little bit to the walls of each pocket.
Let’s revisit two slides we used in Unit 3. The green-and-white molecule is a steroid. The single letter abbreviations represent amino acids coded for by the shuffled genes (for example, Y53H is tyrosine #53 on the heavy chain). The –R groups of these amino acids form the wall of the pocket. Binding of antibody to antigen is so tight that it comes close to the force of covalent binding.
Antibodies are grouped into antibody classes depending on how many Y shapes we have and how they are arranged. Antibody classes are listed in the table: IgG, IgM, IgA, IgE, and IgD. Each of these classes has different functions that we will explore in subsequent objectives.
Antibodies do not destroy anything directly, but they are effective contributors to the overall immune response. Antibody functions include:
- flagging antigens for destruction
- inactivating toxins
- immobilizing bacteria
- activating complement, and enhancing phagocytosis (opsonization)
Media Attributions
- U15-044 Cells of the Immune System © Crookston, Alexa is licensed under a CC BY-NC-ND (Attribution NonCommercial NoDerivatives) license
- U15-045 Macrophage Engulfing Bacterium © Brinkmann, Volker Dr and Planck, Max Institute for Infection Biology, Berlin/ Germany is licensed under a CC BY-NC-ND (Attribution NonCommercial NoDerivatives) license
- U15-046 Phagocytosis © Crookston, Alexa is licensed under a CC BY-NC-ND (Attribution NonCommercial NoDerivatives) license
- U15-047b Chewing Gum © Riddell, Morag is licensed under a CC BY-NC (Attribution NonCommercial) license
- U15-047 Lymphocytes © Crookston, Alexa is licensed under a CC BY-NC-ND (Attribution NonCommercial NoDerivatives) license
- U15-048 T Helper Cell © Crookston, Alexa is licensed under a CC BY-NC-ND (Attribution NonCommercial NoDerivatives) license
- U15-049 T Cytotoxic Cell © Crookston, Alexa is licensed under a CC BY-NC-ND (Attribution NonCommercial NoDerivatives) license
- U15-050 Lymphocyte Function Table © Hutchins, Jim is licensed under a CC BY-SA (Attribution ShareAlike) license
- U15-051 Structure of a B Cell Receptor © Hutchins, jim is licensed under a CC BY-NC-ND (Attribution NonCommercial NoDerivatives) license
- U15-052 B Cell © Crookston, Alexa is licensed under a CC BY-NC-ND (Attribution NonCommercial NoDerivatives) license
- U15-053 Natural Killer Cell © Crookston, Alexa is licensed under a CC BY-NC-ND (Attribution NonCommercial NoDerivatives) license
- U15-047 Ninjaaa © un amigo is licensed under a CC0 (Creative Commons Zero) license
- U15-055 Cluster of Differentiation © Lokal_Profil is licensed under a CC BY-SA (Attribution ShareAlike) license
- U15-056 T Cell Receptor MHC © Goodsell, David is licensed under a CC BY (Attribution) license
- U15-005 Hematopoietic Growth Factors © Häggström, Mikael M.D. is licensed under a CC BY-SA (Attribution ShareAlike) license
- U15-057 Growth Factors Table © Hutchins, Jim is licensed under a CC BY-SA (Attribution ShareAlike) license
- U15-058 Variable Region of an Antibody Binds to the Antigen © Hutchins, Jim; Iwasaki, Akiko and Kim, Sally is licensed under a CC BY-NC-ND (Attribution NonCommercial NoDerivatives) license
- U15-059 Antigen Recognition by Antibodies © Iwasaki, Akiko and Lee, Jung-Hee is licensed under a CC BY-NC-ND (Attribution NonCommercial NoDerivatives) license
- U15-060 Organization and Expression of Immunoglobulin Ig Genes © Feederle, Regina and Schepers, Aloys is licensed under a CC BY-NC-ND (Attribution NonCommercial NoDerivatives) license
- U15-061 Pockets © Hutchins, Jim is licensed under a CC BY-NC-ND (Attribution NonCommercial NoDerivatives) license
- U15-062 Antibody Class Table © Price, Travis is licensed under a CC BY-SA (Attribution ShareAlike) license
- U15-063 Antibody Action Table © Price, Travis is licensed under a CC BY-SA (Attribution ShareAlike) license
- U15-064 Immunoglobulin (IgM) Antibodies Binding to Adjacent Antigen Epitopes © SA1590 is licensed under a CC BY-SA (Attribution ShareAlike) license
- U15-065 Antibody Action © Crookston, Alexa is licensed under a CC BY-NC-ND (Attribution NonCommercial NoDerivatives) license